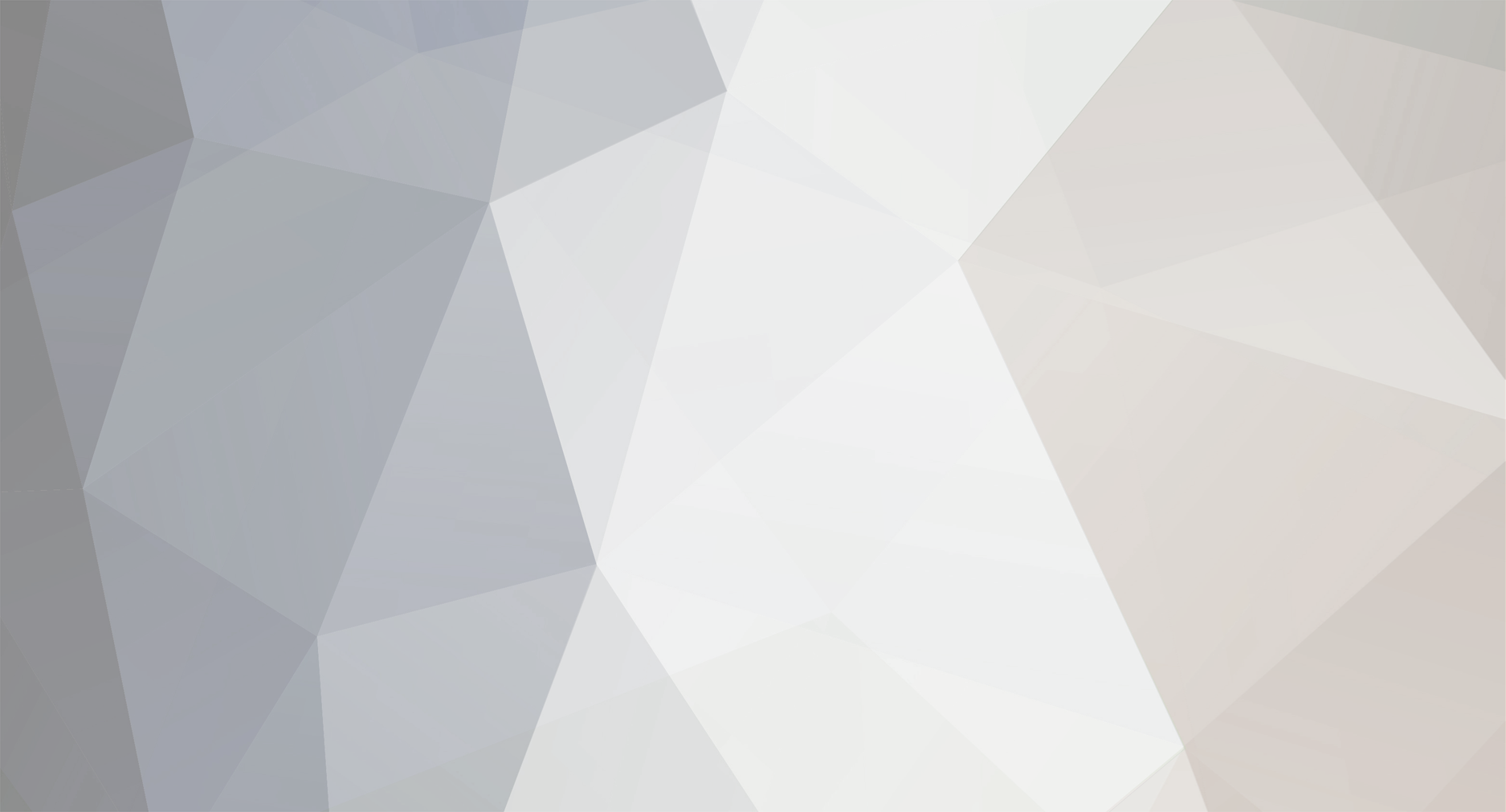
Ace844
Members-
Posts
1,350 -
Joined
-
Last visited
Content Type
Profiles
Articles
Forums
Gallery
Downloads
Store
Everything posted by Ace844
-
The most likely rationale I could think of for the pulse ox still working was the patient was still utilizing compensatory mechanisims in the periphery. Another thing which wasn't mentioned was they could have placed the 'probe' centrally and gotten a reading as well... Not sure, just food for thought & my .02. ACE844
-
I knew you would like that.... :shock: 8)
-
Yes I remember them, and there are other threads here which cover their use. ACE
-
For Rich and Poor, the Message Is Still “Dial 9-1-1”: But Is
Ace844 replied to Ace844's topic in General EMS Discussion
"AZCEP," Anecdotally I know soemone who works in europe in EMS and I spent some time there doing calls this spring while on vacation. I can say the following anecdotally; I do know that there are similar systems in Europe to Canadas which experience the same problems we are seeing here. Also they are experiencing some of the same 'costs' issues we have as well. So it is not merely a US phenomna. Out Here, ACE844 -
Economic Value of Out-of-Hospital Emergency Care: A Structur
Ace844 replied to Ace844's topic in General EMS Discussion
I thought this was a very interesting study as it made plain a number of issues including some of the ones you mention. Additional issues and areas that we need to look ta are as follows. A.) We need to work to get a 'fair' reimbersement and billing system which covers the costs actually incurred during pt care. There was a big push a couple of years ago to get the ICD-9 system set up with an EMS variant. The issue here is that they continuously lower the billing and re-imbursemnet thus decreasing the ability of systems to cover costs. B.) The issue you mention which is pooint of care fees. Until we get our profession, well professional standardized and recognized there is no way other health care providers will sit by and let us take a fair share of the billing pie. I do agree though in EMS we are essential physician extenders, and should be compensated as such. C.) With the scope of practice and higher rates of tort and care increasing how long will it be before we all see our malpractice insurance rates climb without the ability for providers and agencies to 'bill' to cover this? There are more but I will stop here for now, what do you all think? Out Here, ACE844 -
The importance of what is said around patients
Ace844 replied to docomd600's topic in General EMS Discussion
There is another thread here about this started recently by STCommodore I believe...Yes, you must be careful with what you say and when you say it. ACE844 -
Clinical neurophysiology INTRODUCTION — The brain normally produces low voltage electrical activity. This can be measured via the electroencephalogram (EEG), which is ordinarily recorded from the scalp with small surface electrodes. The precise origin of this electrical activity is unknown, but the prevailing belief is that most of the activity represents dendritic synaptic potentials in cortical pyramidal cells [1]. Since there are normal EEG recordings characteristic of certain ages and states of consciousness, it is possible to recognize generalized malfunction of the brain with this modality, as well as localized or paroxysmal abnormalities. The body also produces electrical activity outside the central nervous system, which can be measured in health and disease. Such measurements, performed via peripheral nerve conduction studies, electromyelograms, and neuromuscular function studies, can be used for diagnostic and prognostic purposes. A review of clinical neurophysiology is presented here. Detailed discussions of the use of the various modalities described in this topic review are presented separately in topic reviews relating to specific diseases. ELECTROENCEPHALOGRAM — With the EEG, electrical activity is recorded from many different standard sites on the scalp according to the international (10 to 20) electrode placement system (show figure 1). The nasopharyngeal lead is a long electrode that is passed through the nose and rests on the back of the throat near the mesial aspect of the temporal lobe [2]. Since the typical EEG machine has 16 to 20 channels, a series of different 16 to 20 groups of electrode pairs are evaluated. Recording electrical activity requires measurement of voltage between two electrode sites. It is impossible to record from all possible pairs of electrodes at the same time. There are two different styles of recording: In the referential method (previously referred to as "monopolar"), a series of different electrodes are all referred to the same electrode (eg, the "references"), which is presumed to be relatively electrically inactive (this is similar to the limb leads of an electrocardiogram). Commonly used references are the ears (A1 and A2), vertex (C2) or a non-cephalic reference, such as a "balanced neck-chest" system. With the bipolar method (which utilizes leads that are similar to the chest leads of an electrocardiogram), a series of electrodes in a line are recorded serially as successive pairs. The first recording would be from the first and second electrodes, and the second recording would be from the second and third electrodes, and so on. Use of the different montages given various view of the electrical activity at different parts of the brain. The electrical activity from any electrode pair can be described in terms of amplitude and frequency. Amplitude ranges from 5 µV to 200 µV. Frequency of EEG activity ranges from 0 Hz to approximately 20 Hz. The frequencies are described by Greek letters: Delta — 0 to 4 Hz Theta — 4 to 8 Hz Alpha — 8 to 12 Hz Beta — More than 12 Hz Normal EEG findings — In the normal awake adult with eyes closed, there is a prominent alpha rhythm observed in the posterior part of the head (show figure 2). The amplitude of the alpha falls off anteriorly, which is frequently replaced by some low voltage beta activity. Often, a little low voltage theta activity is observed in fronto-central or temporal regions. The alpha rhythm disappears (or blocks) when the eyes open. This rhythm (which is prominently posterior, blocks eye opening, and is usually in the alpha frequency range) is frequently called the alpha rhythm. However, since the alpha rhythm occasionally may not be in the alpha frequency range and some alpha frequency activity in the EEG may not be the alpha rhythm, semantic confusion sometimes arises concerning the term "alpha." Thus, "alpha" has been associated with alertness and normality of level of consciousness. With drowsiness (which is stage I sleep), the alpha rhythm gradually disappears, fronto-central beta activity may become more prominent and fronto-central-temporal theta activity becomes most significant. As sleep becomes deeper, high voltage single or complex theta or delta waves, which are called vertex sharp waves, appear centrally. Stage II sleep is characterized by increased numbers of vertex sharp waves and centrally predominant runs of sinusoidal 12 to 14 Hz activity called sleep spindles occur. Deeper sleep, characterized by progressively more and higher voltage theta and delta activity, is not usually seen in routine EEG recordings. In routine EEG studies, some "activations" are employed to bring out abnormalities not apparent in the record without such stimulations. These include the following: Three minutes of hyperventilation Flashing a strobe light at different frequencies Drugs, particularly short-acting barbiturates. These can be administered in certain circumstances, particularly to activate epileptic activity (eg, Brevital-EEG) (show figure 3) [3]. Abnormal EEG findings — Abnormalities of the EEG are either focal (only one area of the brain) or generalized (whole brain). Additional abnormalities are either continuous or intermittent. An abnormality that suddenly appears and disappears is called paroxysmal. The accurate interpretation of EEGs requires significant experience. There are a wide variety of normal and variant wave forms as well as artifacts that must be recognized. Slow wave abnormalities — Increased slow activity, meaning the presence of theta and delta activity, in a recording performed when awake is virtually always abnormal. Focal delta activity is usually irregular in configuration and is termed polymorphic delta activity (PDA) (show figure 4). PDA is usually indicative of a focal brain lesion. Another type of delta activity is intermittent, frontally-predominant, and rhythmic; this is called FIRDA (frontal intermittent rhythmic delta activity) (show figure 5), and is indicative of increased intracranial pressure in young people. It is a less specific sign of brain abnormality in the elderly. Encephalopathy — Generalized theta and delta activity is a sign of an encephalopathy. As a general rule, EEG is a sensitive test for encephalopathies. Different patterns of slow wave are associated with different types of encephalopathies [4]. In the general practice of medicine, the evaluation of encephalopathy is the most important use of the EEG, rather than diagnosis of seizure disorders. The most common pattern with metabolic encephalopathies is generalized slowing with moderate or high wave amplitude (show figure 6) [4]. One frequent pattern observed with hepatic and renal encephalopathies, which is sometimes mistaken for epileptogenic activity, is the so-called "liver waves" or "triphasic delta waves" (show figure 7) [5]. With hypoxic encephalopathies, different degrees of slow waves with usually very low amplitudes are observed [6]. Increased beta activity is frequently due to a sedative drug or any centrally active compound, including most depressants, neuroleptics, benzodiazepines, or even alcohol and "illicit" substances (show figure 3). In general, a toxic encephalopathy is indicated by generalized slowing and beta activity. With infectious encephalopathies, there is an admixture of slow activity and the presence of epileptogenic activity as discussed below. In herpes encephalitis, an EEG pattern is identified, which is referred to as periodic lateralized epileptic discharges or PLEDs (show figure 8). (See "Herpes simplex virus type 1 encephalitis"). Epileptogenic abnormalities — EEG is particularly useful in the analysis of seizure disorders. Paroxysmal abnormalities are common between overt seizures (interictally) as well as during seizures (ictally). Such abnormalities include spike and sharp waves: A spike is a single wave that stands out from background activity and has a duration of less than 80 milliseconds. A sharp wave is similar to a spike but has a duration of more than 80 milliseconds. A spike or sharp wave is often followed by a slow wave, and spikes and slow waves can alternate at frequencies from 2 to 5 Hz. Epileptic paroxysmal abnormalities can be generalized or focal. The classic generalized abnormality is the 3 Hz spike and wave pattern which underlies the petit mal absence attack in children (show figure 9). A typical focal abnormality is a focal single spike followed by a slow wave (show figure 10). This abnormality can be seen in focal epilepsy or secondary generalized epilepsies. Occasionally, the abnormal activity spreads to the other hemisphere. If the abnormal electrical activity spreads rapidly to the entire brain, the EEG will be indistinguishable from that of a generalized seizure (show figure 11) [7,8]. Activations, such as hyperventilation, photic stimulation, sleep, sleep deprivation, and drugs, are useful in inducing epileptic activity. Since it is possible to miss infrequent epileptic activity, multiple recordings are frequently helpful. The relationship of any of these abnormalities to the particular patient is complex. As an example, the presence of paroxysmal activity may or may not mean that the problem is related to epilepsy; the final determination may well rest upon the overall clinical picture or the results of a therapeutic trial. The clinician should resist the temptation to consider the results of an EEG independent of other clinical information. In particular, a normal EEG does not exclude epilepsy, since (to cite an extreme case) the EEG may be normal during a focal seizure that is observed clinically. Test characteristics — The sensitivity and specificity of EEGs in seizure disorders vary widely across studies. A meta-analysis of 25 studies found that specificity ranged from 0.13 to 0.99 and sensitivity varied from 0.20 to 0.91 due mainly to large interreader variation [9]. EVOKED POTENTIALS — A stimulus in any sensory modality, whether visual, auditory, or somatosensory, produces a change in the EEG. However, compared to the background EEG, the change is usually small in magnitude, with the exact configuration critically dependent upon the nature of the stimulus and the site of recording on the scalp. The evoked potential is also dependent upon and time-locked to the stimulus, and, in order to see it, the stimulus must be repeated many times and the EEG averaged (show figure 12) [2,10,11]. Evoked potentials can be utilized to test the integrity of a pathway in the CNS. The most common current use of evoked potentials is to test the speed of conduction in a particular pathway. As an example, multiple sclerosis is a disease of central myelin; if myelin is damaged, conduction is slow and evoked potentials are delayed. Since many multiple sclerosis plaques are clinically silent but can be detected with this electrical test, evoked potentials are quite useful in making the diagnosis of multiple sclerosis [12]. (See "Diagnosis of multiple sclerosis"). Visual evoked potentials — Visual evoked potentials (VEPs), which were the first to become popular, are ordinarily obtained by repetitively alternating the black and white squares of a checkerboard. Each eye is stimulated individually and responses are measured from the occipital area of the scalp. Normally, the major wave is a large positive wave at about 100 millisecond latency. The wave is delayed with multiple sclerosis or optic neuritis (show figure 13). Such slowing is not specific for multiple sclerosis. Other conditions include ocular conditions (such as glaucoma), compressive lesions of the optic nerve (such as pituitary lesions), and pathological conditions of the optic radiations or occipital cortex. Auditory evoked potentials — Auditory stimulation produces very complex waveforms. Stimulation with brief clicks produces six small waves in the first 10 milliseconds. The sources of this electrical activity are in serial ascending structures in the brain stem. Thus, since it is possible to study the integrity of the brain stem with these waves, the test has been used to assess "brain stem death" in cases suspected of "brain death" [10,13]. These waves may also be delayed in multiple sclerosis (show figure 14). Somatosensory evoked potentials — Somatosensory evoked potentials (SEPs) are the averaged electrical responses in the central nervous system to somatosensory stimulation. As with sensory nerve action potentials (SNAPs) in the peripheral nervous system (see below), most components of SEPs represent activity carried in the large sensory fibers of the dorsal column, medial lemniscus — primary sensorimotor cortex pathway. Testing the speed of conduction in this pathway permits an assessment of the integrity of the somatosensory system. SEPs from the upper extremity are commonly produced by stimulation of the median nerve at the wrist and are best recorded from a site on the contralateral parietal area. The cerebral SEP to this type of stimulation was the first EP to be discovered. SEPs from the lower extremity are produced by stimulation of the posterior tibial nerve at the ankle or the peroneal nerve at the fibular head; they are recorded best at the vertex of the head (show figure 15). It is possible to localize a lesion in the somatosensory pathway by using short latency SEPs from subcortical structures. Although several systems of electrode placement can be used, the one that appears to produce potentials of greatest amplitude is based upon an active electrode placed over the cervical spine and an "inactive" reference site, such as vertex of the head. There are four components that can be identified, all negative peaks, which are referred to as N9, N11, N13, and N14 [14]: The N9 component clearly originates from the brachial plexus and is best recorded with electrodes placed directly over the plexus itself. The N13 component, which originates from the dorsal column nuclei, is the largest component and is occasionally the only peak identified. By stimulating leg nerves, it is possible to obtain EPs at all levels of the neuraxis, including those over the spinal cord. PERIPHERAL NERVE CONDUCTION STUDIES — A number of modalities are used to evaluate the integrity of peripheral nerves. These principally include sensory and motor nerve conduction studies [15]. Sensory nerve conduction — The cell bodies of sensory neurons are located in the dorsal root ganglia. Each neuron has a central process entering the spinal cord through the dorsal horn and a peripheral process connecting to a sensory receptor in the skin or deep tissues of the limb. The receptors transduce somatosensory stimuli into electrical potentials that eventually give rise to action potentials in the axons; these are transmitted along the peripheral process to the central process. This is called a sensory nerve action potential (SNAPs). These studies can be done orthodromically in the direction of conduction or antidromically in the distal part of major peripheral nerves (show figure 16). There are a variety of functional types of sensory neurons, each with a characteristic spectrum of axonal diameters. Neurons are myelinated or unmyelinated, but the unmyelinated fibers cannot be routinely measured. Many sensory axons with differing function and size coexist in sensory nerves and with motor axons in mixed nerves. The two goals of sensory nerve conduction studies are the assessment of: The number of functioning axons (estimated by measuring the amplitude of SNAP). The state of myelin of these axons (estimated by the conduction velocity of SNAP). With the usual sensory nerve conduction study, all of the axons in a sensory nerve are activated with a pulse of electric current. Action potentials travel along the nerve and the electric field is recorded at a site distant from the site of stimulation. Since each axon makes a contribution to the magnitude of the electrical field, the amplitude of the recorded sensory action potential reflects the number of functioning axons. Utilizing the distance between the site of stimulation and the site of recording and the time between stimulation and the arrival of the action potentials at the recording site, it is possible to calculate a conduction velocity, which reflects the quality of myelination of the axons. In patients with axonal degeneration neuropathies, the primary feature is reduced sensory action potential amplitudes; this can be observed, for example, in diabetic neuropathy. (See "Clinical manifestations and diagnosis of diabetic polyneuropathy"). The conduction velocity may be slightly slowed, but only to the extent that the largest axons are gone; in this setting, the measured conduction velocity reflects the velocity of the largest remaining axons. In addition, slowing of conduction is the primary feature in demyelinating neuropathies, such as Guillain-Barré Syndrome, familial neuropathies, and in compression and entrapment neuropathies, such as carpal tunnel syndrome. (See "Clinical manifestations and diagnosis of carpal tunnel syndrome"). In radiculopathies, both the sensory action potential amplitudes and conduction velocities are normal. This is because the lesion is virtually always proximal to the dorsal root ganglion, and the cell body and its peripheral process remain normal. Sensory action potentials similarly remain normal with lesions of the central nervous system (show figure 17). Motor nerve conduction — There are significant differences between sensory and motor nerve conduction that depend in large part upon differences of anatomy. Motor neurons have cell bodies in the anterior horn of the spinal cord and send their axons to innervate muscle fibers. Motor axons are always intertwined with sensory axons. Unlike pure sensory nerves (eg, the sural nerve), there are no pure motor nerves. Thus, the electrically stimulated compound action potential of any nerve containing motor fibers is really a mixed nerve action potential. It is therefore impossible to deduce the number of functioning motor axons via the measurement of nerve action potential amplitude. However, it is possible to study motor nerve axons separate from sensory axons by electrically stimulating the nerve and recording from the muscle fibers it innervates. The amplitude of the compound muscle action potential is very much larger than the nerve action potential since each motor axon typically innervates hundreds of muscle fibers. The amplitude of the muscle action potential is indicative of the number of activated muscle fibers. As previously mentioned, however, the amplitude is not indicative of the number of motor axons in the nerve. As an example, the number of axons can be diminished and the action potential can remain normal if the process of collateral reinnervation of muscle fibers by the remaining axons has been complete. Conversely, the number of axons can be normal and the action potential diminished if there is diminished synaptic transmission at the neuromuscular junction or if there is loss of muscle fibers. If a neuropathy progresses and collateral reinnervation fails to keep pace, the size of the muscle action potential declines. Motor neuron conduction velocity — Synaptic transmission between nerve and muscle is required to produce a muscle action potential. The delay associated with this process, which is referred to as the distal motor latency, prevents direct calculation of the velocity of motor nerve conduction from a single stimulus location. More specifically, distal motor latency is the total time required for the following events to sequentially occur: The motor nerve action potential to travel down the terminal branches of the axon The release of acetylcholine into the neuromuscular junction, resulting in the end plate potential The generation of a muscle action potential Depending upon the position of the recording electrodes, the time for the muscle action potential to propagate to the recording electrodes Clearly, a calculation of the conduction velocity for this process is not similar to that for sensory nerves. However, if the nerve is stimulated supramaximally in two places, virtually identical muscle action potentials will result; the major difference is the different latencies from the time of stimulation. In turn, the difference in the latencies is due to the difference in the distances from the sites of stimulation to the muscle. Dividing the distances between the two stimulus sites by the difference in the travel times produces a conduction velocity for the segment of nerve between the two sites of stimulation. As with the sensory action potential, measurements of the muscle action potential are ordinarily made to the time of onset; hence, the calculated conduction velocity refers to the fastest (and largest) axons in the nerve (show figure 18). With axonal degeneration neuropathies (the most common variety of generalized neuropathies), motor nerve conduction studies are not significantly abnormal until the process is moderately advanced. Total reliance upon motor nerve conduction with the exclusion of sensory nerve conduction (as practiced in many laboratories) results in the failure to detect many significant neuropathies. There is slight slowing of conduction velocity and prolongation of the distal motor latency to the extent that the largest axons are lost. There may be loss of action potential amplitude when the process is advanced. A focal neurapraxic lesion (eg, carpal tunnel or other compression or entrapment neuropathies) leads to slowing of conduction and decrement of amplitude across the segment including the lesion. However, studies of the nerve distal to the lesion are fully normal. Studies of nerve segments proximal to the lesion reveal normal conduction velocity with an unchanging reduced action potential amplitude. Quite dramatic nerve conduction findings are seen with a focal total neurapraxic lesion (eg, acute trauma or laceration). In this setting, although the nerve is fully normal below the lesion, electrical stimulation proximal to the lesion produces no response (similar to the patients' attempts to activate the muscle). In radiculopathy, motor nerve conduction studies will ordinarily be normal. However, if the process leads to sufficient axonal loss, there may be slight slowing of conduction velocity in direct relation to the amount of loss of large fibers. In central nervous system disease, there will ordinarily be no change in motor nerve conduction unless there is involvement of anterior horn cells. In demyelinating neuropathies, there is slowing of conduction velocity and prolongation of distal motor latency. Late responses — Studying the most proximal segments of nerves is difficult, as the nerves are deep and not easily accessible as they leave the spinal column. Nevertheless, it is useful to study the proximal segments of a nerve, since processes such as radiculopathies from disc protrusion and certain neuropathies such as Guillain-Barré affect this segment predominantly. The so-called late responses, the H-reflex and the F-response, provide a relatively easy technique for the study of the proximal segments of nerves. These responses are produced in certain circumstances after an electrical stimulus to a peripheral nerve and are "late" with respect to the muscle response (the M-response) produced by the orthodromic volley of action potentials traveling to the muscle directly from the electrical stimulus. H-reflex — The H-reflex is a monosynaptic reflex response that is similar in pathway to the tendon jerk. The electrical stimulus activates the Ia afferents (coming from the muscle spindles) and action potentials travel orthodromically to the spinal cord. In the cord, the Ia afferents make excitatory monosynaptic connections to the alpha motor neurons and a volley of action potentials is set up in the motor nerve that runs orthodromically the entire length of the nerve from the cell bodies to the muscle. Thus, action potentials travel through the proximal segment of the nerve twice in the production of the H-reflex, once in the sensory portion of the nerve and once in the motor portion. Obtaining an H-reflex depends upon the ability to stimulate the Ia afferents without stimulating the motor axons. If motor axons are electrically stimulated, an action potential travels along the axon antidromically toward the spinal cord as well as orthodromically toward the muscle. The antidromic action potential collides (either in the proximal motor axon or cell body) with the developing H-reflex in that axon, thereby nullifying it. In routine clinical practice, it is possible to get this differential stimulation and produce H-reflexes only by stimulating the posterior tibial division of the sciatic nerve and recording from the triceps surae. F-response — The F-response, or F-wave, has an advantage over the H-reflexes since it can be found in most muscles. The response is a manifestation of recurrent firing of an anterior horn cell after it has been invaded by an antidromic action potential. After a motor nerve is stimulated, an action potential runs antidromically as well as orthodromically; a small percentage of anterior horn cells that have been invaded antidromically produce an orthodromic action potential that is responsible for the F-response. Thus, to produce an F-response, an action potential must travel twice through the proximal segment of the motor nerve [16]. ELECTROMYOGRAPHY — The physiology of electromyography (EMG) is based upon the function of the motor unit [17]. A motor unit is composed of all the muscle fibers innervated by a single anterior horn cell. In most proximal limb muscles, there are hundreds of fibers in each motor unit. In healthy tissue, muscle fibers from the same unit are not clumped together, but are intermingled with fibers from other motor units. When a motor axon fires, each muscle fiber in its motor unit is activated in a constant time relationship to the other fibers in the unit. EMG activity is ordinarily recorded with a needle placed into the muscle. Because the muscle fibers of a single motor unit are not packed close together, the EMG needle records from only about 10 fibers from each motor unit. The amplitude, duration, and configuration of the electrical activity recorded from a motor unit varies as the needle changes its orientation to the muscle fibers. Despite this variability, it is possible to specify a normal range for the amplitude, duration, and configuration of motor unit action potentials (MUAPs) for each muscle and each age. In relation to configuration, the number of phases above and below the baseline are counted. More than four phases is polyphasic and only a small percentage of polyphasic units in a muscle is considered normal. When an EMG needle is placed in a normal muscle at rest, there is no electrical activity. With weak effort, first one and then several motor units are activated. At this low level of activation, it is possible to see the individual MUAPs and evaluate their parameters. With maximal effort, individual MUAPs cannot be discerned since a large number of units are brought into action. All that can be observed is a dense electrical pattern, called the interference pattern, which can be characterized by its density and peak-to-peak amplitude. The normal density would be either "full" if there are no gaps or "high mixed" if there are a few short gaps. Some patients are unable or unwilling to exert a maximal effort and the pattern will be less dense as a result. Hence, the degree of effort has to be taken into account when assessing the interference pattern. (show figure 19) Electromyographic findings — Findings in EMG are best understood by describing results in different settings. Acute partial axonotmestic injury — Acute partial axonotmestic injury describes a partial laceration of a nerve. The injured motor axons undergo Wallerian degeneration over the course of about five days. Muscle fibers previously innervated by those axons are left denervated. Within approximately 10 to 14 days, denervated muscle fibers develop spontaneous activity. These spontaneous muscle fiber action potentials are recorded by the EMG needle as fibrillations and positive sharp waves. Fibrillations and positive sharp waves are the same except for a slight difference in the particulars of the recording: both are simply small, diphasic potentials that begin with a positive phase (show figure 19). The motor units that can be activated are normal. However, it is not possible to voluntarily activate the denervated muscle fibers. Thus, the interference pattern is less than full and the amplitude is decreased in proportion to the extent of the injury. Descriptive terminology for these patterns, in order of decreasing density, include high mixed, mixed, low mixed, and single unit. After weeks to months, there will be collateral sprouting from surviving motor axons to innervate the denervated muscle fibers. Spontaneous activity will therefore cease. Since motor units contain more muscle fibers than normal, MUAPs will be long in duration, high in amplitude, and more complex in shape or polyphasic. The interference pattern may improve in density, but are likely to remain less than full (although the amplitude increases). Complete neurapraxic injury — With complete neurapraxic injury (or complete laceration), no voluntarily initiated motor nerve action potentials can reach the muscle due to a focal demyelinating injury. However, the axons are intact so that the muscle fibers will not be denervated, and they will not fibrillate. EMG examination do not reveal spontaneous activity, MUAPs, or an interference pattern. This finding is not different from the first few days of a total axonotmestic injury. However, it is significantly different from total axonotmestic injury after the first few days, a time when denervated muscle fibers begin to fibrillate. Myopathy — The simple model of myopathy is characterized by dropout of individual muscle fibers from their motor units. In active myopathies, especially polymyositis, there may be some segmental muscle necrosis. This process divides a muscle fiber into an innervated segment and an uninnervated segment. Since an uninnervated segment may fibrillate, some fibrillation and positive sharp waves may be present in active myopathies, although spontaneous activity is most commonly lacking (show figure 19). (See "Differential diagnosis of peripheral nerve and muscle disease" and see "Approach to the patient with muscle weakness"). NEUROMUSCULAR JUNCTION STUDIES — The most common abnormality at the neuromuscular junction is myasthenia gravis (MG) [17]. Other disorders include the Eaton-Lambert syndrome. (See "Clinical manifestations of myasthenia gravis"). Repetitive nerve stimulation study — A standard neurophysiological study for MG is the repetitive nerve stimulation study or Jolly test. The set-up is similar to that of a motor nerve conduction study. The nerve is stimulated and the muscle action potential is monitored. Normally, the action potential is the same each time; with MG, however, the action potential amplitude may decline on successive stimulations with rates of stimulation of 2 to 10 Hz. There are a variety of patterns that can be observed, but a good measure of this decline is a comparison of the amplitudes of the first and fourth potentials. A decline of more than 10 percent is considered pathologic (show figure 20). In the inverse myasthenic syndrome or Eaton-Lambert syndrome, the Jolly test shows a progressive increase in the amplitude of the muscle action potential. In this setting, rates of 10 to 20 Hz are needed and a potentiation of 200 percent or more is required for the test to be considered pathologic. (See "Paraneoplastic syndromes affecting peripheral nerve and muscle"). Single fiber EMG — Single fiber EMG is sensitive for the diagnosis of MG [18]. In this test, a small needle is used that can detect the action potentials from a pair of muscle fibers of the same motor unit. The time between the firing of two muscle fibers from the same motor unit should be very stable. Due to the slow and uncertain function of the neuromuscular junction with MG, there may be an increase in the variability of the time between filling of these two muscle fibers. This is called increased jitter. (See "Diagnosis of myasthenia gravis"). REFERENCES 1. Schaul, N. The fundamental neural mechanisms of electroencephalography. Electroencephalogr Clin Neurophysiol 1998; 106:101. 2. American Electroencephalographic society. Guidelines in electroencephalography, evoked potentials, and polysomnography. J Clin Neurophysiol 1994; 11:1. 3. Niedermeyer, E, Lopes da Silva, F. Electroencephalography: Basic principles, clinical applications, and related fields, third edition, Williams and Wilkins, Baltimore 1993. 4. Markand, ON. Electroencephalography in diffuse encephalopathies. J Clin Neurophysiol 1984; 1:357. 5. Fisch, BJ, Klass, DW. The diagnostic specificity of triphasic patterns. Electroencephalogr Clin Neurophysiol 1988; 70:1. 6. Synek, VM. Prognostically important EEG coma patterns in diffuse anoxic and traumatic encephalopathies in adults. J Clin Neurophysiol 1988; 5:161. 7. Gotman, J, Marciani, MG. Elelectoencephographic spiking activity, drug levels, and seizure occurrence in epileptic patients. Ann Neurol 1985; 17:597. 8. Goodin, DS, Aminoff, M, Laxer, K. Detection of epileptiform activity by different non-invasive EEG methods in complex partial epilepsy. Ann Neurol 1990; 27:330. 9. Gilbert, DL, Sethuraman, G, Kotagal, U, Buncher, CR. Meta-analysis of EEG test performance shows wide variation among studies. Neurology 2003; 60:564. 10. Chiappa, KH. Evoked Potentials in Clinical Medicine, 3rd ed, Lippincott, New York 1997. 11. Nuwer, MR. Fundamentals of evoked potentials and common clinical applications today. Electroencephalogr Clin Neurophysiol 1998; 106:142. 12. Khoshbin, S, Hallet, M. Multimodality evoked potentials and blink reflex in multiple sclerosis. Neurology 1981; 31:138. 13. Stockard, JJ, Pope-Stockard, JE, Sharbrough, FW. Brainstem auditory evoked potentials in neurology: Methodology, interpretation and clinical applications. In: Electrodiagnosis in clinical neurology, 3rd ed, Aminoff, AM (Ed), Churchill-Livingstone, New York 1992. p.503. 14. Jones, SJ. Short latency potentials recorded from the neck and scalp following median nerve stimulation in man. Electroencephalogr Clin Neurophysiol 1977; 43:853. 15. Aminoff, MJ. Electrodiagnosis in Clinical Neurology, Churchill Livingstone, New York 1998. 16. Anderson, H, Stalnberg, E, Falck, B. F-wave latency, the most sensitive conduction parameter in patients with diabetes mellitus. Muscle Nerve 1997; 20:1296. 17. Preston, DC, Shapiro, BE. Electromyography and Neuromuscular Disorders, Butterworth-Heinemann, Boston 1998. 18. Stalberg, E, Trontelj, JV. Single Fiber Myography, Raven Press, New York 1994.
-
Pharmacology of antiepileptic drugs INTRODUCTION — The rapid increase in the cellular theories of epileptogenesis and the recent availability of second-generation antiepileptic drugs (felbamate, gabapentin, lamotrigine, topiramate, tiagabine, levetiracetam, oxcarbazepine, zonisamide) have resulted in the development of correlations between the in vitro cellular action of antiepileptic drugs (AEDs) and the types of human seizures against which they are most effective [1]. As an example, both carbamazepine and phenytoin inhibit voltage-dependent neuronal sodium channels; this action is predictive of efficacy against tonic seizures. Growing understanding of these correlations has led to the current era of AED development and highlights the importance of understanding the basic mechanisms of AEDs [2,3]. With the exception of felbamate, second generation AEDs (eg, gabapentin, lamotrigine, topiramate, levetiracetam, oxcarbazepine, zonisamide) have a number of potential advantages over older AEDs (eg, phenobarbital, phenytoin, carbamazepine, valproate). These include generally lower side-effect rates, little or no need for serum monitoring, once or twice daily dosing for some, and fewer drug interactions. However, these drugs are not a panacea. The National Institute for Clinical Excellence (UK) practice guidance evaluated newer AEDs (excluding zonisamide and felbamate) and made the following observations [4,5]: Monotherapy data in newly diagnosed epilepsy patients do not show significant differences in effectiveness between older and newer AEDs. Insufficient evidence exists to support the claim that newer AEDs are generally associated with an improved quality of life. There is a high degree of uncertainty about the costs and benefits associated with individual drugs. Freedom from seizures, the desired outcome, is infrequently achieved with combination therapy in patients who have failed monotherapy; insufficient evidence exists to determine whether any of the newer AEDs is superior to other AEDs in providing long-term freedom from seizures. The pharmacology of AEDs is reviewed here. Their use in a treatment plan for patients with seizures is discussed separately. (See "Overview of the management of epilepsy in adults"). DRUGS THAT BLOCK VOLTAGE-DEPENDENT SODIUM CHANNELS — Depolarization of neuronal membranes (such as by excitatory neurotransmitters at postsynaptic receptor sites) produces an influx of sodium ions from the extracellular space into the neuron through sodium channels along the neuronal membrane. As the cell membrane depolarizes, this influx further increases the number of open sodium channels in the cell membrane, further increasing the incoming current of sodium ions. Thus, these sodium channels are termed voltage-dependent. The sodium channels are termed resting or closed when the cell is at resting membrane potential. During the process of depolarization, the sodium channels are activated or open. After activation, they are inactivated (closed) or refractory. Sodium channels cannot be further activated by depolarization during the refractory state. Thus, the membrane potential starts to repolarize and the sodium channels eventually resume the resting or closed state. The inactivated period is usually brief enough to allow for rapid, high-frequency action potentials. Blocking voltage-dependent sodium channels (eg, with tetrodotoxin from the puffer fish), or extending their inactivated state (eg, with certain AEDs), prevents neurons from firing rapidly, although single action potentials are unaffected. This inhibition also can prevent post-tetanic potentiation, a phenomenon consisting of an unusually large response of a postsynaptic neuron to an incoming single action potential after previous exposure to rapid, high-frequency (tetanic) incoming stimulation. Neurons that undergo post-tetanic potentiation may be responsible for spreading abnormal electrical responses after being exposed to intermittently rapid firing neurons. This action may correlate with the rapid spread of epileptic activity from a circumscribed seizure focus to other cortical areas. Carbamazepine — Carbamazepine (CBZ) has been used to treat partial and generalized seizures since being introduced in Switzerland and the United Kingdom over 35 years ago. It is also effective for the treatment of affective illnesses such as bipolar disorder and chronic pain syndromes such as trigeminal neuralgia [6,7]. Carbamazepine is approximately 70 percent protein bound. It is metabolized in the liver by the cytochrome P-450 (CYP) system, an inducible enzyme. The main metabolite, carbamazepine epoxide, has anticonvulsant activity and can be measured in the serum. Carbamazepine binds to voltage-dependent sodium channels, probably after they change from the activated to the inactivated state. This binding extends the inactivated phase and inhibits the generation of rapid action potentials when the cell is experiencing incoming depolarizing trains [8]. The effectiveness of CBZ in inhibiting action potentials by extending the inactivated phase of sodium channel function increases with the rate of neuronal firing. Carbamazepine is used for the initial therapy of primary generalized tonic-clonic seizures and for partial seizures, with or without secondary generalization (show table 1). The usual initial starting dose is 2 to 3 mg/kg per day given twice, three, or four times daily; the dose is increased every five days to 10 mg/kg daily. Generally, three times daily dosing is recommended. However, if patients experience side effects two to four hours after a dose, then the total daily dosage could be redistributed over four doses. Further increases up to 15 to 20 mg/kg per day may be necessary after two to three months because of hepatic autoinduction of the cytochrome P-450 enzyme. Extended-release formulations allow for twice daily dosing with more stable blood levels. Two open-label studies found that switching from immediate-release to extended-release formulation decreased side effects [9,10]. The larger of these two studies (453 patients) also showed improved seizure control [9]. Serum CBZ levels should be measured initially at three, six, and nine weeks, with a goal level of 4 to 12 mcg/mL. Frequent levels are needed early in therapy due to autoinduction, which results in decreased serum concentrations. Serum levels subsequently should be checked at least every two months until successive determinations are constant, more frequently if CBZ dosages or concomitant antiepileptic drug doses are changed. A number of drugs can influence the serum concentration of carbamazepine (show table 2 and show table 3). Common systemic side effects of carbamazepine include nausea, vomiting, diarrhea, hyponatremia, rash, pruritus, and fluid retention (show table 4A-4B). Men with localization-related epilepsy taking carbamazepine have higher rates of sexual dysfunction and low testosterone levels [11]. Carbamazepine has been associated with the Stevens-Johnson syndrome and toxic epidermal necrolysis, particularly during the first eight weeks of therapy [12]. Neurotoxic side effects include drowsiness, dizziness, blurred or double vision, lethargy, and headache. Hyponatremia related to carbamazepine and oxcarbazepine is discussed separately. (See "Hyponatremia" below). Leukopenia occurs in approximately 12 percent of children and 7 percent of adults with CBZ treatment [13]. It may be transient or persistent and does not usually require immediate discontinuation of CBZ therapy [14,15]. The onset is typically within the first three months of treatment. Patients who have a low or low-normal white blood cell (WBC) or neutrophil count before CBZ treatment may be at higher risk for developing CBZ-induced leukopenia or neutropenia. Aplastic anemia (pancytopenia) is a rare, idiosyncratic, non-dose-related side effect that is most likely to occur within the first three or four months after initiating CBZ therapy [14]. Daily laboratory checks would be necessary to monitor for aplastic anemia, agranulocytosis, and thrombocytopenia because of their rapid onset [13], and such frequent monitoring is neither practical nor necessary for most patients taking CBZ. A more suitable approach is to monitor for aplastic anemia by informing patients and physicians to carefully watch for signs and symptoms [13]. (See "Aplastic anemia: Pathogenesis; clinical manifestations; and diagnosis"). Some experts recommend monitoring WBC counts of high-risk patients during the first three months of CBZ treatment, with the monitoring frequency determined by results of each laboratory value. White blood cell counts less than 3000/microliter or neutrophil counts below 1000/microliter warrant either a decrease in CBZ dose with frequent WBC monitoring, or CBZ discontinuation [13]. Phenytoin — Phenytoin was introduced nearly 60 years ago for use in epilepsy and is still widely prescribed for partial and generalized seizures [16]. Similar to carbamazepine, it blocks voltage-dependent neuronal sodium channels [17]. Other effects of phenytoin include diminishing synaptic transmission, limiting fluctuation of neuronal ionic gradients via sodium-potassium ATPase, and affecting second messenger systems by inhibiting calcium-calmodulin protein phosphorylation [18,19]. The first step in the metabolism of phenytoin, which takes place in the liver, involves arene oxidase, which has nonlinear kinetics. In addition to partial and generalized seizures, phenytoin is a second line agent for patients with mixed seizures (myoclonic and tonic-clonic) (show table 1). It is administered orally or intravenously; the latter, or the prodrug fosphenytoin, can be used in patients who are having active seizures. The initial oral dose is 15 mg/kg in three divided doses, followed by a maintenance dose of 5 mg/kg daily in one or two divided doses. Initial blood levels should be obtained two to three weeks after the first dose with a goal concentration of 10 to 20 mcg/mL in patients with normal renal function. In the presence of low serum albumin or other highly protein bound drugs (such as valproate), free levels should be followed with a goal of 1 to 2 mcg/mL. After an oral or intravenous loading dose, the initial phenytoin blood level can be drawn several hours after the conclusion of the loading dose. The results can be used to guide the determination of the maintenance dose or the need for additional loading. Given the long half-life of phenytoin, serum levels should always be checked within five to seven days following any change (increase or decrease) in the daily dose in order to determine the steady-state serum concentration at the new maintenance dose. Like all AEDs, phenytoin dosing should be guided primarily by effect (ie, seizure control) and tolerability. Most, but not all, patients who have normal renal function and serum albumin levels can achieve seizure freedom without side effects with a serum phenytoin concentration of 10 to 20 mcg/mL. A number of drugs can influence the serum concentration of phenytoin (show table 2 and show table 3). Renal failure impairs the protein-binding of phenytoin; the pharmacologically active free concentration may increase relative to the total concentration. Commercially available brand and generic phenytoin products may differ in phenytoin content and other formulation characteristics that can affect bioavailability [20]. These differences may occasionally result in an increase [21] or decrease [22-24] in serum phenytoin levels, which in turn might adversely affect seizure control or cause toxicity when patients are switched from one preparation to another. Therefore, more frequent serum levels and heightened clinical vigilance may be warranted when substituting phenytoin formulations in patients with difficult-to-control seizures or those prone to side effects, particularly in light of phenytoin's nonlinear kinetics and relatively narrow therapeutic window. The major systemic side effects of phenytoin are gingival hypertrophy, body hair increase, rash, and lymphadenopathy (show table 4A-4B). Like carbamazepine, phenytoin has been associated with the Stevens-Johnson syndrome and toxic epidermal necrolysis, particularly during the first eight weeks of therapy [12]. Age-related sexual dysfunction and low testosterone levels are more common in men taking phenytoin than in controls [11]. Neurotoxic side effects include confusion, slurred speech, double vision, ataxia, and neuropathy (with long-term use). Lamotrigine — The cellular mechanism of action of lamotrigine (LTG) is not completely understood; in rodent brain preparations, LTG blocks the repetitive firing of neurons by inactivating voltage-dependent sodium channels (show table 1). However, there is some evidence that LTG, unlike carbamazepine and phenytoin, may selectively influence neurons that synthesize glutamate and aspartate, since it diminishes the release of these excitatory neurotransmitters [25]. These findings suggest that the anticonvulsant effect of LTG may relate to actions on synaptic as well as membrane functions. Lamotrigine is efficacious for the treatment of partial seizures in adults and children (show table 1) [26-28]. It also has demonstrated efficacy as adjunctive therapy in primary generalized tonic clonic seizures [29]. Guidelines issued by the American Academy of Neurology (AAN) state that LTG can be used as initial therapy in patients with newly diagnosed partial epilepsy and idiopathic generalized epilepsy, as well as mixed seizure disorders [26]. Lamotrigine may also be used for the treatment of newly diagnosed absence seizures in children [26]. However, LTG is not approved by the US Food and Drug Administration (FDA) for these indications. Lamotrigine is quickly and totally absorbed when given orally, and plasma concentrations have an apparent linear relationship to dose. The drug is approximately 55 percent bound to plasma proteins, and the liver metabolizes LTG to inactive glucuronide conjugates excreted in the urine. Serum concentrations of LTG are not influenced by interactions with most non-AEDs. However, drug levels are markedly increased by an interaction with valproate, which inhibits glucuronidation, the main metabolic pathway of LTG (show table 3). Levels are decreased in the presence of enzyme-inducing drugs (including phenytoin, carbamazepine, and to a lesser extent oxcarbazepine) [30]. This interaction leads to two different dosing schemes: For patients taking an AED that induces hepatic enzymes (eg, carbamazepine, phenytoin, or mysoline), the initial dose is 25 mg twice daily, titrated upward by 5 mg increments every one to two weeks as needed. For patients taking valproate, the initial dose is 12.5 to 25 mg every other day, with increases of 25 mg every two weeks as needed to a maximum of 300 to 500 mg per day. Levels of LTG can also be influenced by concomitant oral contraceptive agents that decrease levels. This can result in increased concentrations during the "placebo" week used with many preparations, with decreases when the active drug is resumed. This effect appears to be limited to contraceptives containing ethinyl estradiol; progesterone only compounds have not been found to alter LTG levels [31]. Therapeutic serum levels of LTG have not been definitively established. However, data from 811 patients who took LTG as monotherapy or adjunctive therapy revealed a significant correlation between LTG serum concentrations and clinical toxicity [32]. Toxicity was defined as any side effect that required a dose decrease or discontinuation of LTG. The following observations were made. Toxicity increased with increasing serum LTG levels; 7 percent of patients developed toxicity at <5.0 mcg/mL compared with 59 percent at >20 mcg/mL. Toxicity was uncommon at the most frequently encountered serum concentrations (<10 microgram/mL). To put this in perspective, the highest LTG level encountered in any of the major LTG clinical trials was 8.8 mcg/mL, and most patients in those trials had LTG levels in the low single digits (1.53 to 3.60 mcg/mL) [32]. Thus, the authors of this study suggest an initial target range of 1.5 to 10 mcg/mL for LTG therapy, while noting that efficacy may increase at higher levels for patients with refractory seizures. Several studies suggest that LTG clearance increases by about 65 percent in pregnant women with epilepsy, which may lead to an increase in seizures [33,34]. Because of this, frequent monitoring of LTG serum levels and appropriate dose adjustments are advised during the period of withdrawal from oral contraceptives, during pregnancy, and after delivery. Serum monitoring before, during, and after pregnancy can help to guide dosing to avoid a significant decrease during pregnancy or an overshoot due to rebound of levels after delivery. Lamotrigine is excreted in breast milk and may lead to significant serum levels in breast fed infants [35]. Systemic side effects of LTG include rash and nausea (show table 4A-4B). A benign rash may develop in up to 10 percent of patients during the initial one to two months of therapy and necessitates discontinuation of the drug. Patients who have previously had a rash with another AED are more likely to experience rash with LTG [36]. The risk of developing a life-threatening rash such as Stevens-Johnson syndrome, toxic epidermal necrolysis, or angioedema is approximately 1 in 1000 adults; this risk is increased dramatically in children, leading to the recommendation that the drug not be used in patients under the age of 16 years. Neurotoxic side effects are predominantly dizziness and somnolence. In rare cases, lamotrigine has exacerbated or initiated myoclonus and even myoclonic status in juvenile myoclonic and other idiopathic generalized epilepsies [37]. This disappears with withdrawal of the medication and sometimes with lowering the dose. The risk of this side effect is low, and lamotrigine is still considered a treatment option in these patients. Oxcarbazepine — Oxcarbazepine is a compound with a similar chemical structure to carbamazepine and likely a similar mechanism of action (show table 1) [38]. The efficacy of oxcarbazepine is comparable to carbamazepine and other first line therapies for partial and secondarily generalized tonic clonic seizures (show table 1) [26,27,38-40]. However, it is considerably more expensive than the older drugs [41]. Oxcarbazepine is almost completely absorbed regardless of food intake. Serum concentrations of its active metabolite, 10-monohydroxy metabolite (MHD), reach a peak in four to six hours, with a half-life of 8 to 10 hours [38]. The half-life does not change significantly with chronic administration due to a lack of autoinduction. The concentration of this metabolite decreases during pregnancy and increases after delivery [42]. This, with the observation that oxcarbazepine monotherapy was associated with increased seizures in one large pregnancy registry, supports close clinical monitoring during pregnancy and after delivery [43]. There are little other published data on this drug in pregnancy. Metabolism of oxcarbazepine occurs in the liver, but only minimally affects the cytochrome P450 system [38]. This represents a major advantage over carbamazepine, particularly in patients who require polytherapy. Monotherapy in adults begins with 300 to 600 mg/day, increasing to a dose of 900 to 3000 mg/day in two or three divided doses. In infants and young children, one study showed that higher maintenance doses (60 mg/kg per day) were significantly more effective then lower doses (10 mg/kg per day) when used as adjunctive therapy for partial seizures [44]. The most common side effects of oxcarbazepine are sedation, headache, dizziness, rash, vertigo, ataxia, nausea, hyponatremia, and diplopia (show table 4A-4B) [38]. Rare but serious dermatological reactions, including Stevens-Johnson syndrome (SJS) and toxic epidermal necrolysis (TEN), have been associated with oxcarbazepine use in both children and adults; the median time of onset for reported cases was 19 days [45]. These reports prompted the manufacturer and US FDA to issue revised labeling with an updated WARNINGS section in April 2005. In addition, rare multiorgan hypersensitivity reactions have occurred in close temporal association (median time to detection 13 days; range 4 to 60 days) to the initiation of oxcarbazepine therapy in both children and adults. Hyponatremia — Hyponatremia associated with oxcarbazepine and carbamazepine is due at least in part to increased responsiveness of collecting tubules to antidiuretic hormone, and it is considered to be one of the forms of the syndrome of inappropriate secretion of antidiuretic hormone (SIADH). (See "Causes of the SIADH", section on Drugs). The incidence of hyponatremia associated with oxcarbazepine therapy may be higher than that seen with carbamazepine [46,47]. The following observations have been made about the incidence of this complication: Hyponatremia occurred in 23 percent of patients in a postmarketing study, but only 1 percent of patients discontinued oxcarbazepine because of hyponatremia [48]. Hyponatremia may be less common in children than in adults [49,50]. Serum sodium values below 125 meq/L emerged during the first months in 3 percent of patients treated with oxcarbazepine in clinical trials [51]. A cross-sectional analysis of an epilepsy patient database found a prevalence of 12.4 percent for sodium levels less than 128 meq/L among patients taking oxcarbazepine [47]. Elderly patients, particularly those on concomitant natriuretic drugs, are significantly more likely to develop hyponatremia [47,52]. Hyponatremia typically develops gradually in the first few months of therapy, which may explain why most patients are asymptomatic [53]. Evidence for a dose relationship between oxcarbazepine and hyponatremia is conflicting [47,54,55]. Although symptoms attributable to hyponatremia are to some degree related to the severity of the abnormality, the rate of onset is of primary importance. Acute hyponatremia can cause cerebral edema, which can lead to encephalopathy and seizures. Because of a cerebral adaptation, the degree of cerebral edema is less with chronic hyponatremia, and most patients seem to be asymptomatic. (See "Manifestations of hyponatremia and hypernatremia"). Oxcarbazepine-related hyponatremia tends to be mild, asymptomatic, and reversible [48,53], and overall tolerability of oxcarbazepine appears to be better than that of carbamazepine. There is no need to measure the serum sodium concentration at baseline or during oxcarbazepine or carbamazepine therapy unless the patient has some other predisposing factor such as renal failure or a central nervous system lesion that itself causes the syndrome of inappropriate ADH secretion, or the patient has suggestive symptoms [53]. (See "Causes of hyponatremia"). Cessation of oxcarbazepine therapy because of hyponatremia is uncommon, being required in only 1 percent of patients in a postmarketing study. For patients with mild to moderate asymptomatic hyponatremia, the oxcarbazepine can be continued and, if deemed necessary, water restriction and a high salt intake can be initiated in an attempt to raise the serum sodium concentration. (See "Treatment of hyponatremia: SIADH and reset osmostat"). Zonisamide — Zonisamide is a sulfonamide derivative that is chemically and structurally unrelated to other anticonvulsants [56]. Its primary mechanism of action appears to be related to blockage of voltage dependent sodium and T-type calcium channels (show table 1) [57]. Zonisamide is a broad spectrum agent that has been proven effective in randomized, controlled trials as add-on therapy for both partial and generalized seizures (show table 1) [26,27,58-60]. Zonisamide also appears to be effective for myoclonic epilepsy [61,62]. The recommended initial daily dose is 100 to 200 mg per day in two divided doses. Because of its long half-life, once a day dosing is often effective. The dose is increased at two week intervals to a target maintenance dose of 400 to 600 mg per day, although higher doses may be necessary in some patients. The most commonly reported side effects of zonisamide are somnolence, ataxia, anorexia, confusion, abnormal thinking, nervousness, fatigue, and dizziness (show table 4A-4B) [58,59]. Most of these are self-limited, and the likelihood of adverse effects can be reduced by gradually titrating the dose over 4 to 8 weeks [63]. Zonisamide is a weak carbonic anhydrase inhibitor. Nephrolithiasis was reported in 3.7 percent of patients in an early clinical trial [64], but later studies found a much lower risk [3]. (See "Nephrolithiasis in renal tubular acidosis", section on Carbonic anhydrase inhibitors). DRUGS THAT AFFECT CALCIUM CURRENTS — There are three types of calcium channels in neurons, each of which is distinguished by its rate of reactivation and voltage dependency. Low-threshold T-type calcium currents inactivate quickly and have been described in experimental preparations of thalamic relay neurons. These neurons probably are an integral component of the thalamocortical circuits associated with absence seizures, a subtype of generalized seizures associated with brief episodes of staring and a characteristic 3-per-second spike and wave pattern on the electroencephalogram (EEG) [65]. Ethosuximide — Ethosuximide is effective for the treatment of absence seizures; it has no activity against generalized tonic-clonic or partial seizures (show table 1). Ethosuximide diminishes T-type calcium currents in thalamic neurons, which are further reduced as membrane potentials become more hyperpolarized (show table 1) [66]. The metabolite of trimethadione, another AED for absence seizures, acts similarly. The recommended dose of ethosuximide is 20 to 40 mg/day in one to three divided doses. Blood levels should be checked initially after one to three weeks, with a goal therapeutic concentration of 40 to 100 mcg/mL. There are no significant reactions reported with other drugs. The major side effects include nausea, vomiting, sleep disturbance, drowsiness, and hyperactivity (show table 4A-4B). DRUGS THAT AFFECT GABA METABOLISM — Gamma-aminobutyric acid (GABA) is a neurotransmitter that is widely distributed throughout the central nervous system and exerts postsynaptic inhibition. The GABA(A) receptor complex has binding sites for GABA, benzodiazepines, and phenobarbital. Picrotoxin and other similar proconvulsants bind to the GABA(A) receptor and block chloride channels, thereby preventing postsynaptic inhibition. Thus, reduced GABAergic tone is viewed as epileptogenic, while increasing GABAergic tone generally has an anticonvulsant effect [67-69]. Synthesis of GABA is dependent upon the enzyme glutamic acid decarboxylase (GAD), which requires pyridoxine as a coenzyme. Pyridoxine deficient infants lack the capacity to synthesize GABA normally and are prone to seizures. The metabolism of GABA to succinate occurs in presynaptic neurons and glia by means of the mitochondrial enzyme GABA transaminase (GABA-T). Over the past two decades, AEDs have been designed to increase the supply of GABA by lowering GABA metabolism by GABA-T, reducing the reuptake of GABA into neurons and glia, or increasing the production of GABA by GAD. Other AEDs have been designed to imitate the action of GABA, while still others improve endogenous GABA-mediated inhibition (show table 1). Phenobarbital — Phenobarbital is among the oldest AEDs still in use. It is effective for the treatment of generalized and partial seizures (show table 1). However, its clinical utility is limited by its sedating effect (show table 4A-4B). Phenobarbital binds to the GABA(A) receptor, improving the effect of GABA by extending GABA-mediated chloride channel openings [70]. This process permits an increasing flow of chloride ions across the membrane, causing neuronal hyperpolarization (eg, membrane inhibition to depolarization). Benzodiazepines also bind to the GABA(A) receptor and facilitate the attachment of GABA to its binding site on the receptor. The inhibitory action of endogenous GABA is magnified because benzodiazepines increase the occurrence of chloride channel openings. The oral dose of phenobarbital is 1 to 5 mg/kg per day; it may also be administered intravenously. Serum phenobarbital concentrations should be checked three to four weeks after the initial dose, with a goal therapeutic level of 10 to 40 mcg/mL. A number of drugs can influence the serum concentration of phenobarbital (show table 2 and show table 3). Tiagabine — Tiagabine is a second generation AED that is indicated as adjunctive treatment for partial seizures (show table 1) [26,27,71]. It is a potent enhancer of GABA action via specific inhibition of GABA reuptake into presynaptic neurons and glia in vitro (show table 1) [72]. Thus it decreases the elimination of GABA from the synaptic space, making endogenously produced GABA more available for post-synaptic inhibitory effects. The initial dose of tiagabine is 4 to 8 mg/day. It can be titrated in adults at weekly increments of 4 to 8 mg/day until there is a clinical response, or up to 56 mg/day in divided doses. There are no established therapeutic serum levels. Tiagabine has no significant drug interactions. Major side effects include dizziness, lack of energy, somnolence, nausea, nervousness, tremor, difficulty concentrating, and abdominal pain (show table 4A-4B). There is concern that tiagabine has a potential proconvulsive effect. In February 2005, the FDA added a warning label regarding more than 30 reports of seizures, including seven cases of status epilepticus, which were associated with off-label use of tiagabine for patients without epilepsy [73,74]. This issue is not resolved. Tiagabine has been associated with nonconvulsive status epilepticus in patients being treated for localization-related epilepsy in a number of case reports, but this was not observed in randomized, controlled clinical trials or in long-term safety studies [75]. In a retrospective review of patients with localization-related epilepsy, 7.8 percent of 90 tiagabine-treated patients developed nonconvulsive status epilepticus compared with 2.7 percent of the 1165 patients not receiving tiagabine [76]. The frequency of generalized convulsive status epilepticus was not increased. The apparent discrepancy between the risk in seizure and nonseizure patients may be explainable [77]: Tiagabine was developed and is approved for use as an adjunctive treatment for epilepsy. Virtually all patients treated with tiagabine in clinical trials were taking at least one hepatic-enzyme inducing AED, which decreased the concentration of tiagabine; it is likely that patients without epilepsy have increased concentrations of tiagabine. Another potential contributor is the fact that patients taking tiagabine for off-label indications (psychiatric disease and pain) are also on other medications that potentially lower seizure threshold. Vigabatrin — Vigabatrin is an irreversible inhibitor of GABA-transaminase that raises the concentration of GABA in the central nervous system [78]. It is effective as an add-on agent in patients with refractory partial seizures [59,79]. It is also useful as monotherapy [80], although probably less effective that carbamazepine for this purpose [81,82]. Vigabatrin is not available in the United States. It is licensed in Canada and in many countries of Europe and Asia. As many as 30 to 50 percent of patients with long-term exposure to vigabatrin have developed irreversible concentric visual field loss of varying severity that is often asymptomatic [83-87]. In one cohort,this adverse effect was not related to daily dose, duration of exposure, or cumulative dose of vigabatrin [88]. Vigabatrin treatment in laboratory rats is associated with irreversible injury of cone photoreceptors [89]. These findings indicate that vigabatrin should be reserved for patients with partial epilepsy that is refractory to other drugs. Visual field testing should be performed before starting therapy and repeated every six months [90]. Other frequent adverse events with vigabatrin include drowsiness, fatigue, headache, and dizziness [81]. Depression and weight gain also have been reported. Benzodiazepines — Benzodiazepines enhance GABA inhibition by increasing the frequency of GABA-mediated chloride channel openings. Clonazepam is most often used as an adjunctive therapy for myoclonic and atonic seizures. Clorazepate, diazepam, and lorazepam are effective for those seizure types as well as for partial and generalized tonic-clonic seizures. The starting dose of clonazepam in adults is 0.5 to 1.0 mg/day with weekly increments of 0.5 to 1.0 mg/day as needed. Lorazepam and diazepam (especially rectal diazepam) are usually used as PRN medications for acute repetitive seizures or status epilepticus. As a class, the benzodiazepines may be associated with the development of tolerance, limiting their usefulness in the chronic treatment of epilepsy [91]. Side effects include sedation, irritability, ataxia, and depression. Sudden discontinuation of benzodiazepines may lead to withdrawal seizures. DRUGS WITH MULTIPLE MECHANISMS OF ACTION — A number of antiepileptic drugs (AEDs) have multiple mechanisms by which they prevent seizures. Valproate — Valproate (valproic acid) is a broad-spectrum AED used alone and in combination for the treatment of generalized and partial seizures (show table 1). It has multiple cellular mechanisms of action consistent with its broad clinical effectiveness [92-94]. Valproate seems to suppress high frequency, repetitive neuronal firing by blocking voltage-dependent sodium channels, but at sites different than carbamazepine and phenytoin. Valproate increases brain gamma-aminobutyric acid (GABA) concentrations at clinically relevant doses, although the basis of this effect is debated. Valproate does not seem to have any direct effects on the GABA-alpha receptor, but GABA release may be enhanced by a presynaptic effect of valproate on GABA-beta receptors. Inhibition of nerve terminal GABA transaminase (GABA-T) probably also increases presynaptic GABA levels. Furthermore, valproate may increase GABA synthesis by activating glutamic acid decarboxylase (GAD). Finally, valproate acts against T-type calcium currents, although this action is weaker than that observed with ethosuximide. Valproate is tightly protein-bound. It is metabolized in the liver by means of several processes involving oxidation and conjugation. The initial dose is 15 mg/kg per day in three divided doses; it may be increased by 5 to 10 mg/kg per day every week as needed. A serum level should be checked one to two weeks after the initial dose; therapeutic concentrations are usually in the 50 to 150 mcg/mL range. A number of drugs affect the serum level of valproate (show table 2 and show table 3). Valproic acid is available in several formulations, listed here with the corresponding US brand name: Valproic acid capsule, as Depakene Valproic acid syrup, as Depakene Divalproex sodium capsule sprinkles, as Depakote Sprinkle Delayed release divalproex sodium tablet, as Depakote Extended release divalproex sodium tablet, as Depakote ER Valproate sodium injection solution, as Depacon Valproate can be delivered by both oral and intravenous (IV) routes. Current prescribing information recommends slow administration of IV valproate (Depacon) over 60 minutes at a rate of 20 mg/minute, or more rapid infusion of single doses up to 15 mg/kg over 5 to 10 minutes at 1.5 to 3 mg/kg per minute. Accumulating evidence suggests that IV valproate can be safely infused at rates up to 6 mg/kg per minute in adults without adverse effects on blood pressure or heart rate [95-98]. Rapid IV loading may be desirable as a way to achieve serum concentrations >100 mcg/mL quickly. Side effects of valproate include weight gain and obesity [99], nausea, vomiting, hair loss, easy bruising, and tremor (show table 4A-4B). In the United States between 1978 and 1998, there were 37 fatalities due to hepatic failure attributed to valproate use. Among those receiving monotherapy, the calculated rate of fatality was 1 per 37,000 [100]. The rate was much higher for children under the age of two years and for children receiving polytherapy. There were no liver-related fatalities reported in patients over the age of 10 years who were receiving monotherapy. Liver function tests should be periodically monitored in patients on valproate. Valproate-related hyperammonemic encephalopathy (VHE) causes lethargy, increased seizures, and rarely coma and death. VHE can occur without abnormalities of liver function tests or elevated serum valproate levels. (See "Valproic acid intoxication", section on VHE). Felbamate — The mechanism of action of felbamate is not well understood. It blocks the channel at the N-methyl-D-aspartate (NMDA) excitatory amino acid receptor and augments GABA function in rat hippocampal neuronal cultures [101]. It is almost totally absorbed by the gastrointestinal tract, approximately 30 percent bound to plasma protein, and metabolized by the hepatic cytochrome P-450 system. Felbamate can be used to treat partial seizures; Felbamate can also be used to treat the Lennox-Gastaut syndrome, a mixed seizure disorder of childhood onset associated with multiple seizure types, slow spike-wave electroencephalograms, mental retardation, and resistance to standard AEDs [102,103]. However, felbamate has been associated with fatal aplastic anemia and hepatic failure (show table 4A-4B). Aplastic anemia may not occur for several months after the start of therapy, may not be reliably detected by routine testing, and may continue to be a risk for patients even after cessation of the drug. (See "Aplastic anemia: Pathogenesis; clinical manifestations; and diagnosis"). Therapeutic blood levels of felbamate have not been established, but patients should have baseline laboratory testing including a complete blood count and liver enzymes. These tests should continue to be monitored every one to two months, and monitoring of the blood counts should continue following cessation of therapy. Felbamate is not recommended for first-line therapy of seizures because of the potential for serious adverse reactions; use of this drug is mostly confined to cases of Lennox-Gastaut syndrome [104]. The manufacturer recommends that written consent be obtained prior to beginning therapy. Topiramate — Topiramate also has multiple mechanisms of action. It blocks voltage-dependent sodium channels, enhances the activity of GABA at a nonbenzodiazepine site on GABA(A) receptors, and antagonizes an NMDA–glutamate receptor. It also weakly inhibits carbonic anhydrase in the central nervous system [105]. Topiramate is effective as adjunctive therapy for the treatment of adults and children with partial seizures, and may have efficacy for other seizure types (show table 1) [26,27,106,107]. AAN guidelines state that topiramate may be used as initial therapy for newly diagnosed partial and mixed seizure disorders [27]; the guidelines also state that topiramate may be used as monotherapy for refractory generalized tonic-clonic convulsions and partial seizures in adults and children [106]. However, topiramate is not approved by the US Food and Drug administration (FDA) for initial therapy or monotherapy; it is approved for adjunct therapy of partial and primary generalized tonic-clonic seizures. The starting dose of topiramate is 50 mg/day for one week, titrated at weekly increments of 50 mg to an effective dose. The recommended total daily dose for adjunctive therapy is 200 mg twice daily. Therapeutic levels have not been established. Topiramate's clearance is increased twofold by enzyme-inducing agents (eg, phenytoin, carbamazepine), requiring twofold increased dosages in this setting. Topiramate may increase phenytoin concentration, but there do not appear to be any clinically significant interactions with valproate (show table 3). Weight loss is a common dose-related side effect. In a double-blind placebo-controlled trial of topiramate added to existing AEDs in 264 patients, topiramate was associated with a 2.0 kg mean decrease in weight at three months [108]. A smaller, uncontrolled trial found that at one year weight loss occurred in 86 percent with a mean cumulative weight loss of 5.9 kg [109]. Weight loss is associated with fat loss and correlates with reduced caloric intake. Impaired cognition is a reported side effect in a minority of patients taking topiramate, but it is a common reason for discontinuation of therapy [108]. Studies suggest that this may be a more common phenomenon than patient complaints would suggest: In a small, randomized controlled trial of healthy adult volunteers, topiramate monotherapy (target dose 400 mg/day) was associated with significantly impaired test performance on a variety of cognitive measures, including language, compared with baseline [110]. A similar trial in healthy adults showed that the cognitive impairments in topiramate are broad in spectrum [111]. Patients on topiramate scored worse on 38 out of 41 cognitive variables compared with nondrug controls, and all subjects taking topiramate suffered impairment of more than one standard deviation on more than 25 percent of the cognitive variables measured. In a double-blind, randomized cross-over study comparing topiramate and lamotrigine in 29 healthy adults, topiramate but not lamotrigine was associated with slowed responses and errors on tasks of working memory [112]. Other side effects of topiramate include paresthesias, headache, fatigue, dizziness, depression, and mood problems (show table 4A-4B). The incidence of most side effects decrease with continued dosing; weight loss and paresthesia are the exceptions [108]. Despite reports of a high frequency of somnolence when topiramate was used as add-on therapy in patients with epilepsy [113], monotherapy with topiramate 200 mg/day does not appear to impair daytime vigilance in adult patients [114]. Topiramate has been associated with decreased sweating leading to heat intolerance and hyperthermia, particularly in children; there have also been case reports of decreased sweating in adults [115]. Acute myopia and secondary angle glaucoma, characterized by the acute onset of decreased visual acuity and/or ocular pain, also have been reported with topiramate therapy (23 cases out of approximately 825,000 users), typically within one month of initiating treatment [116]. Metabolic acidosis may result from renal bicarbonate loss due to the inhibitory effect of topiramate on carbonic anhydrase, which can cause both proximal and distal acidification defects. The following observations have been made [117,118]: Metabolic acidosis is common, occurring in 32 to 44 percent of adults and 67 percent of children. It is dose-related and usually mild with an average decrease in serum bicarbonate of 4 meq/L at daily doses of topiramate 400 mg in adults and 6 mg/kg per day in children. However, reductions in serum bicarbonate of as much as 10 meq/L have been described. It is most likely to occur early in treatment. The main clinical manifestation of metabolic acidosis is tachypnea, although calcium phosphate nephrolithiasis can occur [119], presumably via a mechanism similar to that seen with other carbonic anhydrase inhibitors such as acetazolamide [120,121] and perhaps zonisamide [3]. It is not known if the risk of nephrolithiasis is increased when two carbonic anhydrase inhibitors are used concurrently. (See "Nephrolithiasis in renal tubular acidosis", section on Carbonic anhydrase inhibitors). Measuring serum bicarbonate at baseline and periodically (for example, every two to four months) is recommended. Gradual dose reduction or cessation of topiramate (after tapering) is advised if significant metabolic acidosis develops. Alkali treatment may be helpful if topiramate is continued in patients with symptoms or more marked acidosis [117]. (See "Treatment of type 1 and type 2 renal tubular acidosis"). Pregabalin — Pregabalin has multiple mechanisms of action. It binds to the alpha2-delta subunit of voltage-gated calcium channels and modulates calcium currents [122,123]. Pregabalin also modulates the release of several neurotransmitters including glutamate, noradrenaline, and substance P. The net result of pregabalin's action appears to be inhibition of neuronal excitability [124]. Pregabalin was approved by the European Medicines Agency (EMEA) in July 2004 as adjunctive therapy for partial seizures and for the treatment of peripheral neuropathic pain. Pregabalin has also received FDA approval for the adjunctive treatment of partial seizures in adults. and for neuropathic pain associated with diabetic peripheral neuropathy and postherpetic neuralgia [125]. Pregabalin is chemically related to gabapentin [126]. It is renally excreted virtually unchanged, and it is not hepatically metabolized [124,127]. Pregabalin does not induce or inhibit the cytochrome P450 system. In addition, it does not bind to plasma proteins. Thus, pregabalin does not have significant interactions with other AEDs and is not expected to have pharmacokinetic interactions with other drugs [128]. Pregabalin exhibits linear pharmacokinetics, has a time to maximal plasma drug concentration (Tmax) of about one hour and a plasma half-life (T1/2) of about six hours [127]. Experimental data suggest that the pharmacodynamic half-life (ie, anticonvulsant effect) of pregabalin is longer than the six-hour pharmacokinetic half-life [129]. The Tmax may be delayed to about three hours if the drug is taken with food, but total absorption is not affected by food. Steady state is achieved within 24 to 48 hours. Pregabalin is effective for the adjunctive treatment of partial seizures as demonstrated in randomized controlled trials [130,131]. In a study that tested pregabalin at the highest anticipated total daily dose of 600 mg, 313 patients with medically refractory partial seizures were randomly assigned to 12 weeks of treatment with pregabalin (either 300 mg twice daily or 200 mg three times daily) or placebo [131]. Both pregabalin regimens were significantly more effective in reducing the frequency of partial-onset seizures than placebo (43 and 53 percent reductions versus a 1 percent increase). The starting dose of pregabalin for the treatment of partial seizures is 150 mg daily given with or without food in either two or three divided doses [132]. Pregabalin may be increased to a daily dose of 300 mg after one week and to a maximum daily dose of 600 mg after an additional week, based on patient response and tolerability. The most common side effects with pregabalin in the studies cited above were dizziness, somnolence, and ataxia (show table 4A-4B) [130,131]. Other side effects include weight gain, peripheral edema, blurred or double vision, asthenia, and abnormal thinking (most often impaired concentration). Pregabalin may also cause euphoria and is classified as a schedule V controlled substance. New onset myoclonus has been reported in patients taking pregabalin for epilepsy [133]. DRUGS WITH UNKNOWN MECHANISM OF ACTION — The precise mechanism of action for gabapentin and levetiracetam is unknown. Gabapentin — Gabapentin was designed to cross the blood-brain barrier and mimic the physiologic effects of GABA, but it binds to a previously unknown receptor rather than to any of the known GABA receptors. Gabapentin is used as add-on therapy for refractory partial seizures (show table 1) [27]. The AAN guidelines state that gabapentin may also be used as initial monotherapy in newly diagnosed partial epilepsy [26], although it is not approved by the US Food and Drug administration (FDA) for this indication. Gabapentin is absorbed by means of amino acid transport systems in the gut [134]. The drug does not bind to plasma protein and is not metabolized; it is excreted entirely in the urine, corresponding with the creatinine clearance [135]. The initial dose of gabapentin is 300 mg the first day, 300 mg twice daily the second day, 300 mg three times a day on the third day, and then increased as needed to 1800 mg/day in three divided doses. A rapid initiation schedule, with a starting dose of 900 mg/day, also appears to be well tolerated [136]. There are no established therapeutic serum levels. A major advantage of gabapentin is that it has no significant drug interactions, making it ideal for use in combination with other AEDs. However, it should be taken at least two hours after the use of antacids since concurrent administration decreases the bioavailability of gabapentin. The major side effect is sedation (show table 4A-4B); it appears to have fewer adverse cognitive effects than carbamazepine [137]. Gabapentin has not been approved in the United States for use in children. In studies of six women taking gabapentin during pregnancy, there was evidence of active transplacental transport and accumulation of the drug in the fetus [138]. Gabapentin is also transferred to breast milk. In this limited series, no adverse consequences of gabapentin on the newborn were seen. Levetiracetam — Levetiracetam (LEV) is a broad spectrum AED that has been found effective and is approved for use as add-on therapy for adults with refractory partial seizures (show table 1) [26,27,139,140]. It also appears to be effective as monotherapy in patients with partial seizures [141], and as add-on therapy for patients with generalized seizures [142]. The mechanism of action for levetiracetam is unknown. Levetiracetam is an attractive AED for several reasons [56]: Metabolism is independent of the cytochrome P450 (CYP) system, so that the elimination half-life of levetiracetam is unaffected by concomitant metabolism of other anticonvulsants. Levetiracetam does not act as an inducer of the CYP system; thus, there is little potential for pharmacokinetic interactions with other drugs, such as oral contraceptives, or immunosuppressant drugs commonly used in organ transplantation [143]. Levetiracetam does not require a titration period, and it appears to have a very rapid onset of action as demonstrated by a significant increase in the proportion of patients who achieved seizure-free status on the first day of LEV 500 mg twice daily treatment compared with placebo [144]. The drug is relatively well tolerated. The most common adverse events include fatigue, somnolence, dizziness, and infection (upper respiratory). Most of these are mild to moderate in intensity and most often occur during the initial titration phase (show table 4A-4B). In a postmarketing surveillance study of 373 patients at a single epilepsy center, both the efficacy of levetiracetam and cumulative probability of 74 percent for remaining on levetiracetam at 12 months compared favorably with published data for vigabatrin, lamotrigine, and topiramate [145]. This was corroborated in a larger, multicenter study in which a 58 percent three-year retention rate was estimated [146]. Sedation was the most common side effect of levetiracetam, occurring in 38 patients (10.7 percent), but mood disturbance was not rare (17 patients or 4.8 percent), and was more likely to lead to discontinuation [145]. Psychiatric adverse effects led to discontinuation in an additional nine patients (2.5 percent), including behavioral disturbance in eight and psychosis in one. Treatment of levetiracetam is initiated at 500 mg twice daily. It is titrated by 1000 mg every two weeks, as needed for seizure control, to a maximum dose of 4000 mg daily. REFERENCES 1. French, JA, Dichter, MA, Leppik, IE (Eds). New Antiepileptic Drug Development: Preclinical and Clinical Aspects. Elsevier, Amsterdam 1993. p. 255. 2. Schachter, SC. Review of the mechanisms of action of antiepileptic drugs. CNS Drugs 1995; 4:469. 3. LaRoche, SM, Helmers, SL. The new antiepileptic drugs: scientific review. JAMA 2004; 291:605. 4. Perucca, E. NICE guidance on newer drugs for epilepsy in adults. BMJ 2004; 328:1273. 5. National Institute for Clinical Excellence (UK). Newer drugs for epilepsy in adults, full guidance. Technology Appraisal Guidance 76, Mar. 2004. www.nice.org.uk/TA076guidance (Accessed 3/7/05). 6. Post, RM, Uhde, TW. Treatment of mood disorders with antiepileptic medications: clinical and theoretical implications. Epilepsia 1983; 24 Suppl 2:S97. 7. Rose, FC, Johnson, FN. Carbamazepine in the treatment of non-seizure disorders: trigeminal neuralgia, other painful disorders, and affective disorders. Rev Contemp Pharmacother 1997; 8:123. 8. MacDonald, RL. Antiepileptic drug actions. Epilepsia 1989; 30:S19. 9. Ficker, DM, Privitera, M, Krauss, G, et al. Improved tolerability and efficacy in epilepsy patients with extended-release carbamazepine. Neurology 2005; 65:593. 10. Miller, AD, Krauss, GL, Hamzeh, FM. Improved CNS tolerability following conversion from immediate- to extended-release carbamazepine. Acta Neurol Scand 2004; 109:374. 11. Herzog, AG, Drislane, FW, Schomer, DL, et al. Differential effects of antiepileptic drugs on sexual function and hormones in men with epilepsy. Neurology 2005; 65:1016. 12. Rzany, B, Correia, O, Kelly, JP, et al. Risk of Stevens-Johnson syndrome and toxic epidermal necrolysis during first weeks of antiepileptic therapy: a case-control study. Lancet 1999; 353:2190. 13. Sobotka, JL, Alexander, B, Cook, BL. A review of carbamazepine's hematologic reactions and monitoring recommendations. DICP 1990; 24:1214. 14. Porter, RJ. How to initiate and maintain carbamazepine therapy in children and adults. Epilepsia 1987; 28 Suppl 3:S59. 15. Bertolino, JG. Carbamazepine. What physicians should know about its hematologic effects. Postgrad Med 1990; 88:183. 16. Merritt, HH, Putnam, TJ. Sodium diphenyl hydantoinate in the treatment of convulsive disorders. JAMA 1938; 111:1068. 17. Yaari, Y, Selzer, M, Pincus, J. Phenytoin: mechanisms of its anticonvulsant action. Ann Neurol 1986; 20:171. 18. Ferrendelli, JA, Mathews, GC. Neuropharmacology of antiepileptic medications: mechanisms of action. In: The Treatment of Epilepsy: Principles and Practice, Wyllie, E (Ed), Lea and Febiger, Philadelphia 1993. p.735. 19. Delgado-Escueta, AV, Horan, MP. Phenytoin: biochemical membrane studies. Adv Neurol 1980; 27:377. 20. Soryal, I, Richens, A. Bioavailability and dissolution of proprietary and generic formulations of phenytoin. J Neurol Neurosurg Psychiatry 1992; 55:688. 21. Mikati, M, Bassett, N, Schachter, S. Double-blind randomized study comparing brand-name and generic phenytoin monotherapy. Epilepsia 1992; 33:359. 22. Rosenbaum, DH, Rowan, AJ, Tuchman, L, French, JA. Comparative bioavailability of a generic phenytoin and Dilantin. Epilepsia 1994; 35:656. 23. Borgheini, G. The bioequivalence and therapeutic efficacy of generic versus brand-name psychoactive drugs. Clin Ther 2003; 25:1578. 24. Burkhardt, RT, Leppik, IE, Blesi, K, et al. Lower phenytoin serum levels in persons switched from brand to generic phenytoin. Neurology 2004; 63:1494. 25. Leach, MJ, Marden, CM, Miller, AA. Pharmacological studies on lamotrigine, a novel potential antiepileptic drug: II. Neurochemical studies on the mechanism of action. Epilepsia 1986; 27:490. 26. French, JA, Kanner, AM, Bautista, J, et al. Efficacy and tolerability of the new antiepileptic drugs I: treatment of new onset epilepsy: report of the Therapeutics and Technology Assessment Subcommittee and Quality Standards Subcommittee of the American Academy of Neurology and the American Epilepsy Society. Neurology 2004; 62:1252. 27. French, JA, Kanner, AM, Bautista, J, et al. Efficacy and tolerability of the new antiepileptic drugs II: treatment of refractory epilepsy: report of the Therapeutics and Technology Assessment Subcommittee and Quality Standards Subcommittee of the American Academy of Neurology and the American Epilepsy Society. Neurology 2004; 62:1261. 28. Schachter, SC. Efficacy and safety of lamotrigine, a new anticonvulsant. Today's Therapeutic Trends 1995; 12:135. 29. Biton, V, Sackellares, JC, Vuong, A, et al. Double-blind, placebo-controlled study of lamotrigine in primary generalized tonic-clonic seizures. Neurology 2005; 65:1737. 30. Weintraub, D, Buchsbaum, R, Resor, SR Jr, Hirsch, LJ. Effect of antiepileptic drug comedication on lamotrigine clearance. Arch Neurol 2005; 62:1432. 31. Reimers, A, Helde, G, Brodtkorb, E. Ethinyl estradiol, not progestogens, reduces lamotrigine serum concentrations. Epilepsia 2005; 46:1414. 32. Hirsch, LJ, Weintraub, D, Du, Y, et al. Correlating lamotrigine serum concentrations with tolerability in patients with epilepsy. Neurology 2004; 63:1022. 33. Tran, TA, Leppik, IE, Blesi, K, et al. Lamotrigine clearance during pregnancy. Neurology 2002; 59:251. 34. de Haan, GJ, Edelbroek, P, Segers, J, et al. Gestation-induced changes in lamotrigine pharmacokinetics: a monotherapy study. Neurology 2004; 63:571. 35. Rambeck, B, Specht, U, Wolf, P. Pharmacokinetic interactions of the new antiepileptic drugs. Clin Pharmacokinet 1996; 31:309. 36. Hirsch, LJ, Weintraub, DB, Buchsbaum, R, et al. Predictors of Lamotrigine-associated rash. Epilepsia 2006; 47:318. 37. Crespel, A, Genton, P, Berramdane, M, et al. Lamotrigine associated with exacerbation or de novo myoclonus in idiopathic generalized epilepsies. Neurology 2005; 65:762. 38. Tecoma, ES. Oxcarbazepine. Epilepsia 1999; 40 Suppl 5:S37. 39. Reinikainen, KJ, Keränen, T, Halonen, T, et al. Comparison of oxcarbazepine and carbamazepine: a double-blind study. Epilepsy Res 1987; 1:284. 40. Schachter, S, Vazquez, B, Fisher, RS, et al. Oxcarbazepine: double-blind, randomized, placebo-controlled, monotherapy trial for partial seizures. Neurology 1999; 52:732. 41. Two new drugs for epilepsy. Med Lett Drugs Ther 2000; 42:33. 42. Mazzucchelli, I, Onat, FY, Ozkara, C, et al. Changes in the disposition of oxcarbazepine and its metabolites during pregnancy and the puerperium. Epilepsia 2006; 47:504. 43. Seizure control and treatment in pregnancy: observations from the EURAP epilepsy pregnancy registry. Neurology 2006; 66:354. 44. Pina-Garza, JE, Espinoza, R, Nordli, D, et al. Oxcarbazepine adjunctive therapy in infants and young children with partial seizures. Neurology 2005; 65:1370. 45. U.S. Food and Drug Administration MedWatch. 2005 Medical Product Safety Alerts. Trileptal (oxcarbazepine) Tablets and Oral Solution. Available online at www.fda.gov/medwatch/SAFETY/2005/safety05.htm#triletpal (Accessed 5/6/05). 46. Asconape, JJ. Some common issues in the use of antiepileptic drugs. Semin Neurol 2002; 22:27. 47. Dong, X, Leppik, IE, White, J, Rarick, J. Hyponatremia from oxcarbazepine and carbamazepine. Neurology 2005; 65:1976. 48. Friis, ML, Kristensen, O, Boas, J, et al. Therapeutic experiences with 947 epileptic out-patients in oxcarbazepine treatment. Acta Neurol Scand 1993; 87:224. 49. Gaily, E, Granstrom, ML, Liukkonen, E. Oxcarbazepine in the treatment of early childhood epilepsy. J Child Neurol 1997; 12:496. 50. Glauser, TA, Nigro, M, Sachdeo, R, et al. Adjunctive therapy with oxcarbazepine in children with partial seizures. The Oxcarbazepine Pediatric Study Group. Neurology 2000; 54:2237. 51. Glauser, TA. Oxcarbazepine in the treatment of epilepsy. Pharmacotherapy 2001; 21:904. 52. Kutluay, E, McCague, K, D'Souza, J, Beydoun, A. Safety and tolerability of oxcarbazepine in elderly patients with epilepsy. Epilepsy Behav 2003; 4:175. 53. Schmidt, D, Arroyo, S, Baulac, M, et al. Recommendations on the clinical use of oxcarbazepine in the treatment of epilepsy: a consensus view. Acta Neurol Scand 2001; 104:167. 54. Nielsen, OA, Johannessen, AC, Bardrum, B. Oxcarbazepine-induced hyponatremia, a cross-sectional study. Epilepsy Res 1988; 2:269. 55. Pendlebury, SC, Moses, DK, Eadie, MJ. Hyponatraemia during oxcarbazepine therapy. Hum Toxicol 1989; 8:337. 56. Schachter, SC. The next wave of anticonvulsants. Focus on levetiracetam, oxcarbazepine and zonisamide. CNS Drugs 2000; 14:229. 57. Leppik, IE. Zonisamide. Epilepsia 1999; 40 Suppl 5:S23. 58. Schmidt, D, Jacob, R, Loiseau, P, et al. Zonisamide for add-on treatment of refractory partial epilepsy: a European double-blind trial. Epilepsy Res 1993; 15:67. 59. Marson, AG, Kadir, ZA, Hutton, JL, Chadwick, DW. The new antiepileptic drugs: a systematic review of their efficacy and tolerability. Epilepsia 1997; 38:859. 60. Brodie, MJ, Duncan, R, Vespignani, H, et al. Dose-dependent safety and efficacy of zonisamide: a randomized, double-blind, placebo-controlled study in patients with refractory partial seizures. Epilepsia 2005; 46:31. 61. Henry, TR, Leppik, IE, Gumnit, RJ, Jacobs, M. Progressive myoclonus epilepsy treated with zonisamide. Neurology 1988; 38:928. 62. Kyllerman, M, Ben-Menachem, E. Zonisamide for progressive myoclonus epilepsy: long-term observations in seven patients. Epilepsy Res 1998; 29:109. 63. Bergen, D. Incidence of adverse events is reduced with titrated dosing of Zonegran (zonisamide) for partial seizures in the United States (abstract). Epilepsia 1999; 40 Suppl 7:94. 64. Leppik, IE, Willmore, LJ, Homan, RW, et al. Efficacy and safety of zonisamide: results of a multicenter study. Epilepsy Res 1993; 14:165. 65. Coulter, DA, Huguenard, JR, Prince, DA. Specific petit mal anticonvulsants reduce calcium currents in thalamic neurons. Neurosci Lett 1989; 98:74. 66. Coulter, DA, Huguenard, JR, Prince, DA. Characterization of ethosuximide reduction of low-threshold calcium current in thalamic neurons. Ann Neurol 1989; 25:582. 67. Meldrum, BS. Epilepsy and gamma-aminobutyric acid-mediated inhibition. Int Rev Neurobiol 1975; 17:1. 68. Meldrum, B. GABA-agonists as anti-epileptic agents. Adv Biochem Psychopharmacol 1981; 26:207. 69. Dichter, MA, Ayala, GF. Cellular mechanisms of epilepsy: a status report. Science 1987; 237:157. 70. Twyman, R, Rogers, C, MacDonald, R. Differential regulation of gamma-aminobutyric acid receptor channels by diazepam and phenobarbital. Ann Neurol 1989; 25:213. 71. Schachter, SC. Tiagabine: current status and potential clinical applications. Exp Opin Invest Drugs 1996; 5:1377. 72. Braestrup, C, Nielsen, EB, Sonnewald, U, et al. ®-N-(4,4-bis(3-methyl-2-thienyl)but-3-en-1-yl)nipecotic acid binds with high affinity to the brain GABA uptake carrier. J Neurochem 1990; 54:639. 73. FDA public health advisory: seizure in patients without epilepsy being treated with Gabitril (tiagabine). February 18,2005. (Accessed March 7, 2006, at http://www.fda.gov/cder/drug/advisory/gabitril.htm). 74. The FDA Safety Information and Adverse Event Reporting Program. 2005 Safety Alert: Gabitril (tiagabine HCl): important drug warnging. February 14, 2005. (Accessed March 7, 2006, at http://www.fda.gov/MEDWATCH/SAFETY/2005/gabitril_DHCP.htm). 75. Shinnar, S, Berg, AT, Treiman, DM, et al. Status epilepticus and tiagabine therapy: review of safety data and epidemiologic comparisons. Epilepsia 2001; 42:372. 76. Koepp, MJ, Edwards, M, Collins, J, et al. Status epilepticus and tiagabine therapy revisited. Epilepsia 2005; 46:1625. 77. Flowers, CM, Racoosin, JA, Kortepeter, C. Seizure activity and off-label use of tiagabine. N Engl J Med 2006; 354:773. 78. Petroff, OA, Rothman, DL, Behar, KL, et al. Human brain GABA levels rise rapidly after initiation of vigabatrin therapy. Neurology 1996; 47:1567. 79. Loiseau, P, Hardenberg, JP, Pestre, M, et al. Double-blind, placebo-controlled study of vigabatrin (gamma-vinyl GABA) in drug resistant epilepsy. Epilepsia 1986; 27:115. 80. Tanganelli, P, Regesta, G. Vigabatrin vs. carbamazepine monotherapy in newly diagnosed focal epilepsy: a randomized response conditional cross-over study. Epilepsy Res 1996; 25:257. 81. Chadwick, D, for the Vigabatrin European Monotherapy Study Group. Safety and efficacy of vigabatrin and carbamazepine in newly diagnosed epilepsy: a multicentre randomised double-blind study. Lancet 1999; 354:13. 82. Kälviäinen, R, Äikiä, M, Mervaala, E, et al. Vigabatrin versus carbamazepine monotherapy in newly diagnosed patients with epilepsy. Arch Neurol 1995; 52:989. 83. Eke, T, Talbot, JF, Lawden, MC. Severe persistent visual field constriction associated with vigabatrin. BMJ 1997; 314:180. 84. Kalviainen, R, Nousiainen, I, Mantyjarvi, M, et al. Vigabatrin, a gabaergic antiepileptic drug, causes concentric visual field defects. Neurology 1999; 53:922. 85. Miller, NR, Johnson, MA, Paul, SR, et al. Visual dysfunction in patients receiving vigabatrin: clinical and electrophysiologic findings. Neurology 1999; 53:2082. 86.
-
Status epilepticus in adults INTRODUCTION — Although exact definitions vary, the term status epilepticus generally refers to the occurrence of a single unremitting seizure with a duration longer than 5 to 30 minutes or frequent clinical seizures without an interictal return to the baseline clinical state [1-3]. The diagnostic evaluation and clinical management of status epilepticus will be discussed here. The management of chronic epilepsy, the actions of antiepileptic drugs, and the management of status epilepticus in children are discussed separately. (See "Overview of the management of epilepsy in adults", see "Pharmacology of antiepileptic drugs"and see "Management of status epilepticus in children"). EPIDEMIOLOGY — It is estimated that there are 100,000 to 200,000 episodes of status epilepticus in the United States annually [4]. Refractory status epilepticus, defined as ongoing seizures following first- and second-line drug therapy, was noted in nearly 30 [5] to 43 [6] percent of patients with status epilepticus. Although not associated with increased mortality, refractory status epilepticus was linked to prolonged hospitalization and poorer functional outcomes. Encephalitis was associated significantly more often with refractory than with nonrefractory status epilepticus (22 versus 4 percent, respectively). In contrast, inadequate serum levels of antiepileptic drugs (AEDs) were associated significantly more often with nonrefractory than with refractory status epilepticus (28 versus 0 percent, respectively). Also, generalized tonic-clonic status was less likely to be refractory than nonconvulsive or focal motor status. ETIOLOGY — Optimal management of status epilepticus requires identification and correction, if possible, of any predisposing factors that are present. Virtually any acute or chronic brain injury, as well as a number of toxic-metabolic insults, can cause status epilepticus [1,7]. Some of the more common predisposing factors include: Antiepileptic drug noncompliance or discontinuation Withdrawal syndromes associated with the discontinuation of alcohol, barbiturates, baclofen, or benzodiazepines (particularly alprazolam) Acute structural injury (eg, encephalitis, tumor, stroke, head trauma, subarachnoid hemorrhage, cerebral anoxia or hypoxia) Remote or longstanding structural injury (eg, prior head injury, cerebral palsy, previous neurosurgery, perinatal cerebral ischemia, arteriovenous malformations) Metabolic abnormalities (eg, hypoglycemia, hepatic encephalopathy, uremia, pyridoxine deficiency, hyponatremia, hyperglycemia, hypocalcemia, hypomagnesemia) Use of, or overdose with drugs that lower the seizure threshold (eg, theophylline, imipenem, high dose penicillin G, quinolone antibiotics, metronidazole, isoniazid, tricyclic antidepressants (especially bupropion), lithium, clozapine, flumazenil, cyclosporine, lidocaine, bupivacaine, metrizamide, and, to a lesser extent, phenothiazines) Chronic epilepsy; status epilepticus may represent part of a patient's underlying epileptic syndrome (as with the Landau-Kleffner syndrome or Rasmussen's encephalitis), or may be associated with any of the generalized epilepsies COMPLICATIONS AND OUTCOME — The mortality rate for adults who present with a first episode of status epilepticus is roughly 20 percent [4,8]. Estimates vary widely, mainly as a function of the underlying etiology and whether status epilepticus following cerebral anoxia is included in the study [1,9,10]. The mortality for status epilepticus after anoxia ranges from 69 to 81 percent [4,7,11]. Older age and high initial APACHE-II scores (a prognostic scoring system for ICU patients based on underlying disease, chronic conditions, and physiologic variables) are also independent risk factors for mortality [12]. Many of the other underlying causes of status epilepticus (see "Etiology" above) are associated with significant morbidity and mortality, even in the absence of seizures. In one series, 89 percent of deaths in patients with status epilepticus were attributed to the underlying etiology [13]. Another study suggested that acute symptomatic status is associated with a six-fold increased risk of mortality from status epilepticus [14]. The mortality of status epilepticus is in part due to the metabolic stress of repeated muscular convulsions. Rhabdomyolysis, lactic acidosis, aspiration pneumonitis, neurogenic pulmonary edema, and respiratory failure may complicate convulsions [1]. In addition, cardiac injury due to massive release of catecholamines may also contribute to injury [15,16] (See "Cardiac complications of stroke", section on neurogenic cardiac damage). In addition, neuronal death can occur under certain circumstances after as little as 30 to 60 minutes of continuous seizure activity [17-19]. The pathologic hallmark of this phenomenon, cortical laminar necrosis, may also be seen on brain MRI as a persistant, high intensity lesion on T1-weighted images, which follows the gyral anatomy of the cerebral cortex [20]. Clinically, this effect manifests in increasing neurologic morbitidty with increasing seizure duration, even after the effects of etiology are eliminated [18,21]. Consequently, survivors of status epilepticus are at significant risk for recurrent seizures, and up to 10 percent may be left with disabling neurologic deficits [14]. DIAGNOSIS — The diagnosis of status epilepticus is often straightforward, but may be more complicated when symptoms are continuous and affect focal cognitive functions like language. The diagnosis of status epilepticus rests primarily upon the neurologic examination and the electroencephalogram (EEG). Neurologic examination — Although the diagnosis of tonic-clonic status epilepticus may be obvious, a neurologic examination can be critical in making the diagnosis in more complex cases. Particularly important are assessment of the level of consciousness, observations for automatic movements or myoclonus, and any asymmetric features on examination that may indicate a focal structural lesion. (See "The detailed neurologic examination in adults" and see "Stupor and coma in adults", section on the Neurologic examination). Electroencephalogram — The EEG is an extremely valuable tool in evaluating the patient with suspected status epilepticus. An EEG that reveals continuous seizure activity is diagnostic of status epilepticus. However, there are some limitations to the use of the EEG in this context [22]: Clear ictal activity may not be seen during simple partial status epilepticus Some ictal EEG patterns are difficult to recognize or are controversial An EEG obtained over a short period of time and between seizures can miss intermittent ictal activity Many cases of status epilepticus can be diagnosed on the basis of the neurologic examination alone, but EEG data are invaluable in diagnosing complex cases. (See "Clinical neurophysiology"). Certain EEG patterns in unresponsive patients are diagnostic of status epilepticus, including patterns that show temporal evolution. The meaning of other patterns, such as periodic lateralized epileptiform discharges (PLEDS), remains controversial, although aggressive pharmacologic treatment in a patient whose EEG shows only PLEDS without evolution and whose examination reveals no clinical seizures should generally be avoided [23]. Serial EEG recordings may be helpful if the initial study is not diagnostic. Single photon emission computed tomography — Single photon emission computed tomography (SPECT) may demonstrate areas of increased perfusion during status epilepticus that can persist for weeks after the termination of seizures [24]. SPECT may be helpful in diagnosing status epilepticus when the EEG is equivocal. However, good studies of sensitivity and specificity are not available, and results should be interpreted in conjunction with clinical and EEG findings. Magnetic resonance imaging — Magnetic resonance imaging (MRI) is the best tool to reveal the structural lesions that may trigger status epilepticus, but MRI is not a first-line test for diagnosing status epilepticus. However, MRI may reveal areas of increased signal intensity on FLAIR, T2-, or diffusion-weighted images in the presence of status epilepticus [25,26]. These findings are believed to represent seizure-induced cellular edema and are seen in cortical and limbic structures, particularly the hippocampus [27]. They may persist for days to weeks, especially if seizures are prolonged, and either ultimately resolve or evolve into focal atrophy and sclerosis. These lesions are nonspecific and can be associated with many other processes. CLASSIFICATION — Classifying the type of status epilepticus is important because it is a major factor in determining morbidity and, therefore, the aggressiveness of treatment that is required; generalized tonic-clonic or partial-complex status epilepticus poses the greatest risk. The three most common forms of status epilepticus are: Simple partial — Simple partial status epilepticus is characterized by continuous or repeated focal motor seizures (eg, twitching of one thumb), focal sensory symptoms (eg, the sensation of flashing lights in one visual field), or cognitive symptoms (eg, aphasia) without impaired consciousness. Complex partial — Complex partial status epilepticus is characterized by continuous or repeated episodes of focal motor, sensory, or cognitive symptoms with impaired consciousness, and should be considered in the differential diagnosis of acute confusional states [28]. Other symptoms, such as automatisms and behavioral disturbances, may also occur. Generalized tonic-clonic — Generalized tonic-clonic status epilepticus is always associated with impaired consciousness. Tonic-clonic seizures may be the initial manifestation of status epilepticus, or may represent secondary generalization from other seizure types. Less common varieties — In addition to the three major types of status epilepticus listed above, there are a number of less common but important forms to recognize: Absence — Absence (petit mal) status epilepticus is characterized by altered awareness, but not necessarily unconsciousness. Patients are typically confused or stuporous, and there may be associated myoclonus, eye blinking, perseveration, altered motor performance, language difficulty, or other symptoms. Absence status epilepticus typically occurs in patients with chronic epilepsy and frequently requires EEG for confirmation. Myoclonic — Myoclonic status epilepticus is characterized by frequent myoclonic jerks in the setting of altered mental status. This typically occurs in patients with one of the generalized epilepsies, such as juvenile myoclonic epilepsy. The term has also been applied by some authors to the myoclonus seen in the patient who has experienced global cerebral ischemia. However, this myoclonus should not be considered in the same category as myoclonic status epilepticus unless EEG recordings demonstrate actual seizure activity and not simply a burst-suppression pattern. Overall, patients with myoclonus and altered consciousness are far more likely to be suffering from a metabolic encephalopathy (particularly uremic or hepatic encephalopathy) than from true myoclonic status epilepticus. Psychogenic — Although relatively rare, psychogenic status epilepticus should be considered in situations where there are bilateral motor movements with preserved consciousness. An EEG recording during one of the patient's typical clinical events can help establish this diagnosis, although the EEG may also appear relatively normal during simple partial status epilepticus. (See "Factitious disorder and Munchausen syndrome"). Although prolactin levels drawn shortly after the onset of a seizure may help differentiate epileptic and non-epileptic events, prolactin levels normalize during prolonged seizures and so are not helpful in the diagnosis of status epilepticus [29]. PHARMACOLOGIC AGENTS — There are four main categories of drugs used to treat status epilepticus: benzodiazepines, phenytoin (or fosphenytoin), barbiturates, and propofol [2]. Other treatments with drugs such as lidocaine, paraldehyde (which is no longer available in the United States for intravenous infusion), chloral hydrate, ketamine, carbamazepine, or etomidate are less efficacious or less well studied and should not be considered part of the routine management of status epilepticus. Similarly, general anesthesia with isoflurane or other inhalational agents may be temporarily effective in stopping seizures but is used only in extreme circumstances because of logistical problems. Other agents such as chlormethiazole are used in other countries but are not available in the United States [30]. Benzodiazepines — Benzodiazepines remain the first-line treatment for status epilepticus because they can rapidly control seizures [31]. A number of studies have addressed the different uses and pharmacology of the three most commonly used benzodiazepines for status epilepticus: diazepam, lorazepam, and midazolam. All are thought to increase chloride conductance in central nervous system GABA(A) receptors and thus decrease neuronal excitability [32]. Diazepam — Diazepam has a high lipid solubility and therefore an ability to rapidly cross the blood-brain barrier; it is highly effective in rapidly terminating seizures when administered at doses of 0.1 to 0.3 mg/kg intravenously. An affect upon seizure activity can be seen as early as 10 to 20 seconds after administration, and cerebrospinal fluid (CSF) concentrations reach half of their maximum value in three minutes. However, because of subsequent redistribution of the drug into adipose tissue, the duration of diazepam's acute anticonvulsant effect is typically <20 minutes. Initial termination of seizure activity with intravenous diazepam is seen in 50 to 80 percent of patients [33], but if no other medication is provided, there is a 50 percent chance of seizure recurrence within the next two hours [34,35]. Nonetheless, diazepam remains the drug of first choice in many settings because it is stable in liquid form for long periods at room temperature. Therefore, diazepam is available in resuscitation kits in premixed form, while lorazepam, midazolam, and phenytoin are not. A rectal gel formulation of diazepam is also marketed and provides rapid delivery when intravenous access is problematic. Lorazepam — Although lorazepam is as effective as diazepam in terminating seizures, the time from its injection to its maximum effect against seizures is as long as two minutes. The clinical advantage of lorazepam is that the effective duration of action against seizures is as long as four to six hours because of its less pronounced redistribution into adipose tissue. Effective initial intravenous doses of lorazepam are 0.02 to 0.2 mg/kg. One study randomized 570 patients with a verified diagnosis of status epilepticus to one of four initial regimens [33]: lorazepam (0.1 mg/kg), phenytoin (18 mg/kg), diazepam (0.15 mg/mL) plus phenytoin (18 mg/kg), or phenobarbital (15 mg/kg). In the subgroup of 384 patients with overt generalized convulsive status epilepticus, treatment with lorazepam alone was most effective in terminating seizures within 20 minutes and maintaining a seizure-free state in the first 60 minutes after treatment (65 percent versus 58 percent with phenobarbital, 56 percent with diazepam plus phenytoin, and 44 percent with phenytoin alone). This observation is supported by a Cochrane review of 11 studies encompassing 2017 patients [36]. No significant differences in the success rates of the different regimens were observed in the 134 patients with subtle generalized convulsive status epilepticus, and overall there were no significant differences in seizure recurrence during the 12-hour study period, outcome at 30 days, or in the incidence of adverse events. Midazolam — Like lorazepam and diazepam, midazolam is very effective in acutely terminating seizures (frequently in less than one minute), but it has a short half-life in the central nervous system. The advantage of midazolam over the other two benzodiazepines is that its use as a continuous infusion for refractory status epilepticus has been more thoroughly investigated and is associated with minimal cardiovascular side effects [32]. Effective initial intravenous doses of midazolam are a 0.2 mg/kg bolus, followed by continuous infusion at rates of 0.75 to 10 µg/kg per minute. A continuous midazolam infusion is probably less effective than high dose barbiturates or propofol for the treatment of refractory status epilepticus, although high quality studies directly comparing these treatments have not been performed. Nasally administered midazolam may be useful in the rapid termination of seizures when IV access is difficult [37], but additional studies are needed before this can be recommended. Clonazepam — Clonazepam has been used to treat status epilepticus outside the United States in settings where intravenous formulations are available. It has effects similar to those of other benzodiazepines, with a rapidity of onset that is intermediate between that of lorazepam and that of diazepam. Its duration of activity is more prolonged than that of diazepam. Phenytoin — Phenytoin is one of the most commonly used treatments for status epilepticus, despite the trial described above which showed that initial treatment of generalized convulsive status epilepticus with lorazepam alone was more effective than treatment with diazepam and phenytoin [33]. The principal advantage of phenytoin derives from its efficacy in preventing the recurrence of status epilepticus for extended periods of time. Phenytoin is generally infused at a rate of up to 50 mg/minute to a total dose of 20 mg/kg, but it is critical to modify the infusion rate in the presence of hypotension or other adverse cardiovascular events. The risks of hypotension and cardiac arrhythmias increase with higher infusion rates, partly due to the propylene glycol used to solubilize phenytoin. In addition, the risks of local pain and injury (including venous thrombosis and the purple glove syndrome) increase with more rapid rates of infusion. Cardiac monitoring during the initial infusion is mandatory because bradyarrhythmias or tachyarrhythmias may occur. Fosphenytoin — Fosphenytoin is a pro-drug of phenytoin that is hydrolyzed into phenytoin by serum phosphatases. Fosphenytoin is highly water soluble and therefore unlikely to precipitate during intravenous administration. The risk of local irritation at the site of infusion is significantly reduced compared with phenytoin; fosphenytoin can therefore be infused much more rapidly (up to 150 mg/minute versus 50 mg/minute with phenytoin). In addition, the increased water solubility of fosphenytoin makes intramuscular (IM) administration possible if intravenous (IV) access cannot be obtained. However, IM administration will yield less predictable levels and a longer time to onset of effect than IV administration. Since propylene glycol is not required to solubilize fosphenytoin, the cardiovascular side effects of fosphenytoin may be less frequent and severe than those of phenytoin. However, at least two studies have suggested that the incidence of adverse hemodynamic effects with fosphenytoin and phenytoin infusions is similar [38,39]. Since fosphenytoin is converted on a 1:1 molar basis to phenytoin, the dosing of fosphenytoin in terms of moles is identical. However, the molecular weight of fosphenytoin is greater than that of phenytoin; hence, a greater weight of fosphenytoin must be given in order to yield the same concentration of phenytoin. To eliminate this problem, the manufacturer recommends prescribing of fosphenytoin as milligrams of phenytoin equivalent (PE). Orders for fosphenytoin should be written in terms of PEs; as an example, 20 mg/kg PE load at a rate of 100 to 150 mg PE/minute. Cardiac monitoring is required during the infusion of fosphenytoin or phenytoin. Barbiturates — Barbiturates are similar to benzodiazepines in that they also bind to the GABA(A) receptor, amplifying the actions of GABA by extending GABA-mediated chloride channel openings [40]. This process permits an increasing flow of chloride ions across the membrane, causing neuronal hyperpolarization (eg, membrane inhibition to depolarization). Phenobarbital and pentobarbital are the most useful barbiturates in the treatment of status epilepticus. Phenobarbital — Phenobarbital is an excellent anticonvulsant, especially in the acute stage of managing seizures. Various studies have shown a rate of seizure control of approximately 60 percent when phenobarbital is used alone; this rate is similar to that seen with lorazepam alone or the combination of phenytoin and diazepam [33,41]. Despite its efficacy, phenobarbital is generally not used as a first-line treatment in adults because it carries a higher risk of hypoventilation and hypotension than benzodiazepines or phenytoin. Initial doses of 20 mg/kg infused at a rate of 30 to 50 mg/minute are generally used, but slower infusion rates should be used in the elderly. Careful monitoring of respiratory and cardiac status is mandatory. It is often necessary to intubate patients in order to provide a secure airway and minimize the risk of aspiration if phenobarbital is administered following benzodiazepines. The risk of prolonged sedation with phenobarbital is greater than with the other anticonvulsants because of its half-life of 87 to 100 hours. Pentobarbital — Pentobarbital is used primarily in the treatment of refractory status epilepticus, typically with a loading dose of 10 mg/kg infused at a rate of up to 100 mg/minute [42]. The ultimate infusion rate is determined by the amount of drug required to terminate status epilepticus, but can be limited by hypotension due to the drug's adverse inotropic and vasodilatory effects. Vasopressors are almost universally required during high dose pentobarbital infusions, and pulmonary artery catheterization may be required to optimize volume status and facilitate vasopressor management. (See "Use of vasopressors and inotropes" and see "Swan-Ganz catheterization: Indications and complications"). Thiopental — Some centers use thiopental instead of pentobarbital for refractory status epilepticus, but there are a number of problems with this approach [30]. Animal studies suggest that thiopental carries a higher incidence of adverse cardiovascular effects than pentobarbital. The half-life of thiopental is shorter than that of pentobarbital, but this is counterbalanced by the fact that thiopental is degraded to active metabolites (including pentobarbital), which accumulate with longer term infusions. Thiopental may also have immunosuppressive effects on neutrophil function and mucociliary clearance [43,44]. An uncontrolled series of 10 consecutive adults presenting with status epilepticus noted that high-dose thiopental therapy effectively terminated clinical and electrophysiological evidence of seizures [45]. However, therapy was associated with systemic hypotension; each patient required fluid resuscitation with an average of 2.6 L of crystalloid in the first 24 hours. High-dose thiopental resulted in delayed recovery from anesthesia, and six patients developed S. aureus pneumonia, resulting in prolonged intubation. Propofol — Propofol is a hindered phenolic compound with anticonvulsant properties. The drug is unrelated to any of the currently used barbiturate, opioid, benzodiazepine, arylcyclohexylamine, or imidazole intravenous anesthetic agents. Hypotension and respiratory depression may complicate its use. Experience with propofol in the treatment of status epilepticus is limited, but promising results have been reported in several small trials [18,46,47]. As an example, one study compared the results of treatment with propofol or high dose barbiturates in 16 patients with refractory status epilepticus [48]. Termination of seizures was significantly faster among successfully treated patients in the propofol group (mean 3 versus 123 minutes), but there was a nonsignificant trend toward higher overall success rates in barbiturate-treated patients (82 versus 63 percent). Valproic acid — The use of intravenous (IV) valproic acid (Depacon) in the treatment of status epilepticus has been limited in part because the United States Food and Drug Administration (FDA) approved it only for slow infusion rates (up to 20 mg/min). However, accumulating evidence suggests that more rapid infusion rates and higher intravenous loading doses of valproate are safe and well tolerated. (See "Pharmacology of antiepileptic drugs", section on Valproate). The limited available data suggest that valproate may be useful in treating acute status epilepticus [49-51], but questions remain about the relative effectiveness of IV valproate compared with other antiepileptic drugs (AEDs) that are first-line agents for treating status epilepticus. In addition, the risk of hyperammonemic encephalopathy due to valproate, may pose diagnostic challenges in the postictal setting [52]. (See "Valproic acid intoxication"). Further clinical trial data are needed to define the role of IV valproate in this setting. TREATMENT — Any patient with possible status epilepticus requires rapid evaluation and treatment [53]. There are many possible pharmacologic approaches to status epilepticus; successful approaches to management have been developed empirically, since there are few controlled trials comparing different regimens [2,3]. Although there are differences in efficacy and side effect profile among effective agents, it is important to become familiar with and primarily use one reasonable treatment method. We divide the initial management into three phases: assessment/supportive treatment, initial pharmacologic therapy, and secondary pharmacotherapy (if required) for the treatment of refractory seizures. Assessment and support — A rapid neurologic examination should be performed to provide a preliminary classification of the type of status epilepticus and its probable etiology. The patient should also undergo a rapid general evaluation, with particular attention to respiratory and circulatory status, and supportive therapy (eg, oxygen, mechanical ventilation) should be instituted as needed. During this time, intravenous catheters should be placed and blood obtained for electrolyte, serum glucose, and toxicology studies, a complete blood count, and a rapid "finger-stick" glucose. Measurement of arterial blood gases is often valuable and may suggest a need for intubation and mechanical ventilatory support. Cardiac monitoring, frequent measurement of blood pressure, and pulse oximetry should be instituted. These tasks may require one to four minutes and may overlap with the next phase of treatment. Initial pharmacologic therapy — Lorazepam 0.02 to 0.03 mg/kg should be administered intravenously and approximately one minute allowed to assess its effect. Diazepam 0.1 mg/kg IV or midazolam 0.05 mg/kg IV may be substituted if lorazepam is not available. If seizures continue at this point, additional doses of lorazepam (up to a cumulative dose of 0.1 mg/kg) should be infused at a maximum rate of 2 mg/minute, and a second intravenous catheter placed in order to begin a concomitant phenytoin (or fosphenytoin) loading infusion. Even if seizures terminate after the initial lorazepam dose, therapy with phenytoin or fosphenytoin is generally indicated to prevent the recurrence of seizures. Phenytoin and any of the benzodiazepines are incompatible and will precipitate if infused through the same intravenous line. A phenytoin infusion of 20 mg/kg (or 20 mg/kg phenytoin equivalents (PE) for fosphenytoin) should be started at 25 to 50 mg/min (or 100 mg PE/minute for fosphenytoin) and reduced if significant adverse effects of the infusion are seen. This phase of treatment usually lasts approximately 30 minutes. Treatment of refractory seizures — Status epilepticus that is refractory to first line anticonvulsants indicates a grave prognosis and requires management in an intensive care setting. After failure of the first line therapy discussed above, the next step is to consider infusion of another 10 mg/kg of phenytoin (or 10 mg/kg PE of fosphenytoin) and up to another 0.05 mg/kg of lorazepam if the patient is stable. Metabolic derangements from initial laboratory studies should be appropriately treated. Further measures are required if seizures continue, but whereas there is reasonable agreement upon treatment up to this point, the optimal therapy of refractory status epilepticus is less well defined. Regardless of the specifics of pharmacologic therapy, it is critical to provide adequate ventilatory and hemodynamic support. Patients with refractory seizures should be endotracheally intubated, and continuous electroencephalogram (EEG) recordings are desirable [54]. The primary drugs used for refractory status epilepticus are phenobarbital, pentobarbital, midazolam, and propofol. There is no consensus about which should be used first. A systematic review of drug therapy for refractory status epilepticus assessed data on 193 patients from 28 trials in an attempt to clarify this issue [54]. Overall mortality was 48 percent, but there was no association between drug selection and the risk of death. Pentobarbital was more effective than either propofol or midazolam in preventing breakthrough seizures (12 versus 42 percent), but was associated with a significantly increased incidence of hypotension, defined as a systolic blood pressure below 100 mmHg (77 versus 34 percent). In contrast, another study of 107 patients failed to show an influence of the therapy used on the outcome of refractory status [55]. Further pharmacologic therapy at this point is based primarily upon the patient's hemodynamic stability and the risk for prolonged mechanical ventilation. Hemodynamically stable patients — Treatment with high-dose barbiturates (pentobarbital or phenobarbital) remains common in this setting because of the greatest experience with its use [42]. However, propofol is gaining some acceptance in this setting for patients who are already intubated because response to therapy is very rapid, allowing a rapid change to another regimen if propofol infusion is unsuccessful. Continuous EEG monitoring should be instituted, if possible, along with continuous pulse oximetry and blood pressure monitoring via an arterial catheter. Vasopressors should be available at the bedside. (See "Use of vasopressors and inotropes"). An initial dose of 20 mg/kg of phenobarbital should be infused at a maximum rate of 100 mg/minute. If seizure activity continues, a dose of 10 mg/kg of pentobarbital should be infused while careful attention is paid to the EEG and hemodynamic status. Additional doses of pentobarbital at rates up to 100 mg/min should be infused until seizures stop and the EEG shows a burst-suppression pattern. The primary advantage of the burst-suppression endpoint is that it is easily recognizable, but achieving a burst-suppression pattern is not always necessary [55]. Almost all patients at this point will require vasopressor support (typically phenylephrine or dopamine), as well as crystalloid infusions. The mortality rate associated with barbiturate coma is high because of adverse hemodynamic effects and the severity of the underlying neurologic process, and reaches 80 percent in patients over 70. If seizures are terminated with pentobarbital, then an infusion at 1 to 4 mg/kg per hour should be maintained for 24 hours and tapered over the following 24 hours. Some physicians may prolong the duration of high-dose therapy if frequent focal epileptiform discharges remain on the EEG after treatment. During this time, high therapeutic phenytoin and/or phenobarbital concentrations must be maintained. Hemodynamically unstable patients — Treatment with barbiturates or propofol may significantly worsen the hemodynamics of unstable patients. Therefore, one option is to proceed with a midazolam infusion because it is the best-tolerated treatment in this setting [3,32]. Generally, therapy is initiated with a 0.2 mg/kg bolus, followed by a continuous infusion of 0.05 to 0.5 mg/kg per hour. If this is unsuccessful within 45 to 60 minutes, a propofol or pentobarbital infusion should be started. Patients at high risk for respiratory failure — Patients who are at high risk for prolonged mechanical ventilation (eg, those with severe COPD, severe debilitation, or cancer) should be treated with propofol in an attempt to minimize the duration of sedation [48]. Pressors should be ready at the bedside, and blood pressure and EEG monitored closely while propofol infusion is initiated at 1 to 2 mg/kg per hour. This infusion should be titrated over the next 20 to 60 minutes to maintain a seizure-free state and burst suppression on the EEG. Infusion rates up to 10 to 12 mg/kg/hour may be required. If seizures are controlled with propofol, the effective infusion rate should be maintained for 24 hours and then tapered at a rate of 5 percent per hour. This prevents rebound seizures that commonly occur with abrupt propofol discontinuation. It is critical that high therapeutic levels of at least one anticonvulsant (phenytoin levels >25 mg/L [99 µmol/L] or phenobarbital levels >30 mg/L [129 µmol/L]) are obtained prior to tapering the propofol in order to reduce the risk of seizure recurrence. Treatment with propofol should generally be considered unsuccessful if it does not terminate seizure activity within 45 to 60 minutes. In this case, a high dose barbiturate infusion should be considered. Propofol infusions for refractory status epilepticus are relatively new in comparison with midazolam or high dose barbiturates. However, as clinical experience with propofol sedation in the intensive care setting grows, this agent is increasingly used in patients with refractory status persisting after intubation. It remains critical that propofol be employed cautiously and by individuals familiar with its use in this context. Malignant status epilepticus — The term "malignant" status epilepticus has been introduced to refer to status epilepticus that either fails to respond to the therapies discussed above or recurs quickly on tapering these medications [56]. It has been reported that as many as 20 percent of patients with refractory status epilepticus evolve into malignant status epilepticus, a transition that is associated with a very poor prognosis. Out-of-hospital treatment — Treatment of status epilepticus out of hospital by paramedics appears to be safe and effective. This was illustrated in a randomized, double-blind study of 205 patients with status epilepticus, of whom 66 received lorazepam, 68 received diazepam, and 71 received placebo [57]. Status epilepticus had been terminated on arrival to the emergency department in more patients treated with lorazepam and diazepam than placebo (59, 43, and 21 percent, respectively). Active treatment also reduced the rates of respiratory or circulatory complications (10.6, 10.3, and 22.5 percent, respectively). SUMMARY AND RECOMMENDATIONS — Status epilepticus refers to the occurrence of a single unremitting seizure with a duration longer than 5 to 30 minutes or frequent clinical seizures without an interictal return to the baseline clinical state. Etiologies include noncompliance with antiepileptic drug (AED) treatment, drug or alcohol withdrawal syndromes, acute brain injury or infection, and metabolic disturbances, among others. (See "Etiology" above). The prognosis depends most strongly on the underlying etiology; however, there is some evidence that status epilepticus is independently associated with mortality and neurologic sequelae. (See "Complications and outcome" above). The diagnosis of status epilepticus can be difficult. A careful neurologic examination and EEG studies are important in situations where there is any uncertainty. (See "Diagnosis" above). The initial assessment and treatment of a patient in status epilepticus should proceed relatively simultaneously. Hemodynamic and respiratory monitoring are also required in order to avoid side effects of therapy. (See "Assessment and support" above). We recommend lorazepam 0.02 to 0.03 mg/kg IV as the initial treatment for status epilepticus (Grade 1A). A loading dose of phenytoin or fosphenytoin should follow to maintain anti-seizure effect (Grade 1B). (See "Initial pharmacologic therapy" above). There are many possible approaches to the treatment of status epilepticus. One possible approach is summarized in the accompanying protocol (Grade 2C). (See "Treatment of refractory seizures" above). Clinicians should employ those medication regimens with which they and the care team are familiar in order to avoid unintended complications of therapy. REFERENCES 1. Aminoff, MJ, Simon, RP. Status epilepticus: Causes, clinical features and consequences in 98 patients. Am J Med 1980; 69:657. 2. Treatment of convulsive status epilepticus. Recommendations of the Epilepsy Foundation of America's Working Group on Status Epilepticus. JAMA 1993; 270:854. 3. Lowenstein, DH, Alldredge, BK. Status epilepticus. N Engl J Med 1998; 338:970. 4. DeLorenzo, RJ, Pellock, JM, Towne, AR, Boggs, JG. Epidemiology of status epilepticus. J Clin Neurophysiol 1995; 12:316. 5. Mayer, SA, Claassen, J, Lokin, J, Mendelsohn, F. Refractory Status Epilepticus: Frequency, Risk Factors, and Impact on Outcome. Arch Neurol 2002; 59:205. 6. Holtkamp, M, Othman, J, Buchheim, K, Meierkord, H. Predictors and prognosis of refractory status epilepticus treated in a neurological intensive care unit. J Neurol Neurosurg Psychiatry 2005; 76:534. 7. Towne, AR, Pellock, JM, Ko, D, DeLorenzo, RJ. Determinants of mortality in status epilepticus. Epilepsia 1994; 35:27. 8. Logroscino, G, Hesdorffer, DC, Cascino, G, et al. Short-term mortality after a first episode of status epilepticus. Epilepsia 1997; 38:1344. 9. Hauser, WA. Status epilepticus: Epidemiologic considerations. Neurology 1990; 40(5 suppl 2):9. 10. Chin, RF, Neville, BG, Scott, RC. A systematic review of the epidemiology of status epilepticus. Eur J Neurol 2004; 11:800. 11. Lowenstein, DH, Alldredge, BK. Status epilepticus at an urban public hospital in the 1980s. Neurology 1993; 43:483. 12. Prasad, A, et al. Propofol and midazolam in the treatment of refractory status epilepticus. Epilepsia 2001; 42:380. 13. Shorvon, S. Prognosis and outcome of status epilepticus. In: Status epilepticus: its clinical features and treatment in children and adults, Shorvon, S, (Ed), Cambridge Uinversity Press, Cambridge 1909. p.293. 14. Claassen, J, Lokin, JK, Fitzsimmons, BF, et al. Predictors of functional disability and mortality after status epilepticus. Neurology 2002; 58:139. 15. Manno, EM, Pfeifer, EA, Cascino, GD, et al. Cardiac pathology in status epilepticus. Ann Neurol 2005;58:954. 16. Simon, RP, Aminoff, MJ, Benowitz, NL. Changes in plasma catecholamines after tonic-clonic seizures. Neurology 1984; 34:255. 17. Fountain, NB, Lothman, EW. Pathophysiology of status epilepticus. J Clin Neurophysiol 1995; 12:326. 18. Payne, TA, Bleck, TP. Status epilepticus. Crit Care Clin 1997; 13:17. 19. Chapman, MG, Smith, M, Hirsch, NP. Status epilepticus. Anaesthesia 2001; 56:648. 20. Donaire, A, Carreno, M, Gomez, B, et al. Cortical laminar necrosis related to prolonged focal status epilepticus. J Neurol Neurosurg Psychiatry 2006; 77:104. 21. Scholtes, FB, Renier, WO, Meinardi, H. Generalized convulsive status epilepticus: causes, therapy, and outcome in 346 patients. Epilepsia 1994; 35:1104. 22. Westmoreland, BF. Epileptiform electroencephalographic patterns. Mayo Clin Proc 1996; 71:501. 23. Reiher, J, Rivest, J, Grand'Maison, F, Leduc, CP. Periodic lateralized epileptiform discharges with transitional rhythmic discharges: Association with seizures. Electroencephalogr Clin Neurophysiol 1991; 78:12. 24. Tatum, WO, Alavi, A, Stecker, MM. Technetium-99m-HMPAO SPECT in partial status epilepticus. J Nucl Med 1994; 35:1087. 25. Henry, TR, Drury, I, Brunberg, JA, et al. Focal cerebral magnetic resonance changes associated with partial status epilepticus. Epilepsia 1994; 35:35. 26. Chu, K, Kang, DW, Kim, JY, et al. Diffusion-weighted magnetic resonance imaging in nonconvulsive status epilepticus. Arch Neurol 2001; 58:993. 27. Briellmann, RS, Wellard, RM, Jackson, GD. Seizure-associated abnormalities in epilepsy: evidence from MR imaging. Epilepsia 2005; 46:760. 28. Privitera, M, Hoffman, M, Moore, JL, Jester, D. EEG detection of nontonic-clonic status epilepticus in patients with altered consciousness. Epilepsy Res 1994; 18:155. 29. Chen, DK, So, YT, Fisher, RS. Use of serum prolactin in diagnosing epileptic seizures: report of the Therapeutics and Technology Assessment Subcommittee of the American Academy of Neurology. Neurology 2005; 65:668. 30. Walker, MC, Smith, SJM, Shorvon, SD. The intensive care treatment of convulsive status epilepticus in the UK. Anaesthesia 1995; 50:130. 31. Treiman, DM. Pharmacokinetics and clinical use of benzodiazepines in the management of status epilepticus. Epilepsia 1989; 30(suppl 2):s4. 32. Kumar, A, Bleck, TP. Intravenous midazolam for the treatment of refractory status epilepticus. Crit Care Med 1992; 20:483. 33. Treiman, DM, et al. A Comparison of four treatments for generalized convulsive status epilepticus. N Engl J Med 1998; 339:792. 34. Prensky, AL, Raff, MC, Moore, MJ, Schwab, RS. Intravenous diazepam in the treatment of prolonged seizure activity. N Engl J Med 1967; 276:779. 35. Walker, M. Status epilepticus: an evidence based guide. BMJ 2005; 331:673. 36. Prasad, K, Al-Roomi, K, Krishnan, P, et al. Anticonvulsant therapy for status epilepticus. Cochrane Database Syst Rev 2005; :CD003723. 37. Mahmoudian, T, Zadeh, MM. Comparison of intranasal midazolam with intravenous diazepam for treating acute seizures in children. Epilepsy Behav 2004; 5:253. 38. Coplin, WM, Rhoney, DH, Rebuck, JA, et al. Randomized evaluation of adverse events and length-of-stay with routine emergency department use of phenytoin or fosphenytoin. Neurol Res 2002; 24:842. 39. Swadron, SP, Rudis, MI, Azimian, K, et al. A comparison of phenytoin-loading techniques in the emergency department. Acad Emerg Med 2004; 11:244. 40. Twyman, R, Rogers, C, MacDonald, R. Differential regulation of gamma-aminobutyric acid receptor channels by diazepam and phenobarbital. Ann Neurol 1989; 25:213. 41. Shaner, DM, McCurdy, SA, Herring, MO, et al. Treatment of status epilepticus: A prospective comparison of diazepam and phenytoin versus phenobarbital and optional phenytoin. Neurology 1988; 38:202. 42. Yaffe, K, Lowenstein, DH. Prognostic factors of pentobarbital therapy for refractory generalized status epilepticus. Neurology 1993; 43:895. 43. Kress, HG, Segmuller, R. [intravenous anesthetics and human neutrophil granulocyte motility in vitro]. Anaesthesist 1987; 36:356. 44. Forbes, AR, Gamsu, G. Depression of lung mucociliary dlearance by thiopental and halothane. Anesth Analg 1979; 58:387. 45. Parviainen, I, Uusaro, A, Kalviainen, R, et al. High-dose thiopental in the treatment of refractory status epilepticus in intensive care unit. Neurology 2002; 59:1249. 46. Prasad, A, Worrall, BB, Bertram, EH, Bleck, TP. Propofol and midazolam in the treatment of refractory status epilepticus. Epilepsia 2001; 42:380. 47. Rossetti, AO, Reichhart, MD, Schaller, MD, et al. Propofol treatment of refractory status epilepticus: a study of 31 episodes. Epilepsia 2004; 45:757. 48. Stecker, MM, et al. Treatment of refractory status epilepticus with propofol. Epilepsia 1998; 39:18. 49. Hodges, BM, Mazur, JE. Intravenous valproate in status epilepticus. Ann Pharmacother 2001; 35:1465. 50. Yu, KT, Mills, S, Thompson, N, Cunanan, C. Safety and efficacy of intravenous valproate in pediatric status epilepticus and acute repetitive seizures. Epilepsia 2003; 44:724. 51. Limdi, NA, Shimpi, AV, Faught, E, et al. Efficacy of rapid IV administration of valproic acid for status epilepticus. Neurology 2005; 64:353. 52. Rossetti, AO, Bromfield, EB. Efficacy of rapid IV administration of valproic acid for status epilepticus. Neurology 2005; 65:500. 53. Marik, PE, Varon, J. The management of status epilepticus. Chest 2004; 126:582. 54. Claassen, J, Hirsch, LJ, Emerson, RG, Mayer, SA. Treatment of refractory status epilepticus with pentobarbital, propofol, or midazolam: a systematic review. Epilepsia 2002; 43:146. 55. Rossetti, AO, Logroscino, G, Bromfield, EB. Refractory status epilepticus: effect of treatment aggressiveness on prognosis. Arch Neurol 2005; 62:1698. 56. Holtkamp, M, Othman, J, Buchheim, K, et al. A "malignant" variant of status epilepticus. Arch Neurol 2005; 62:1428. 57. Alldredge, BK, Gelb, AM, Isaacs, SM, et al. A comparison of lorazepam, diazepam, and placebo for the treatment of out-of-hospital status epilepticus. N Engl J Med 2001; 345:631
-
Evaluation of the first seizure in adults INTRODUCTION — A seizure is a sudden change in behavior that is the consequence of brain dysfunction. Epileptic seizures result from electrical hypersynchronization of neuronal networks in the cerebral cortex. Epilepsy is characterized by recurrent epileptic seizures due to a genetically determined or acquired brain disorder [1]. Approximately 0.5 to 1 percent of the population has epilepsy. Nonepileptic seizures (NES), are sudden changes in behavior that resemble epileptic seizures but are not associated with the typical neurophysiological changes that characterize epileptic seizures [2-4]. NES are subdivided into two major types: physiological and psychogenic. Physiological NES are caused by a sudden alteration of neuronal function due to metabolic derangement or hypoxemia. Causes of physiological NES include cardiac arrhythmias, syncope, and severe hypoglycemia. Psychogenic NES are thought to result from stressful psychological conflicts or major emotional trauma and are more challenging to recognize and diagnose than physiological NES, but rarely occur de novo in patients without a significant psychiatric history. The pharmacological treatment of epileptic seizures is directed at restoring neuronal function to normal, while the treatment of NES is specific to the disorder that triggered the seizure. Thus, the primary goal in evaluating a patient's first seizure is to resolve whether the seizure resulted from a treatable systemic process or intrinsic dysfunction of the central nervous system and, if the latter, the nature of the underlying brain pathology. This evaluation will determine the likelihood that a patient will have additional seizures and assist in the decision whether to begin anticonvulsant therapy [5,6]. The differential diagnosis and clinical features of seizures and the diagnostic evaluation of the first seizure in adults are reviewed here. While convulsive and nonconvulsive status epilepticus may be the initial presentation of epilepsy, they are not specifically discussed because clinical recognition is straightforward [7]. (See "Status epilepticus in adults"). The treatment of chronic epilepsy is reviewed separately. (See "Overview of the management of epilepsy in adults"). ETIOLOGY Epileptic seizures — Less than one-half of epilepsy cases have an identifiable cause. It is presumed that epilepsy in most, if not all, of these other patients is genetically determined. In the remainder of patients in whom an etiology can be determined, the causes of epileptic seizures include congenital brain malformations, inborn errors of metabolism, high fevers, head trauma, brain tumors, stroke, intracranial infection, cerebral degeneration, withdrawal states, and iatrogenic drug reactions [8]. (See "Post-traumatic seizures and epilepsy"). In the elderly, vascular, degenerative, and neoplastic etiologies are more common than in younger adults and children [9]. A higher proportion of epilepsy in children is due to congenital brain malformations than in other age groups. (See "Epilepsy syndromes in children"). These general principles were illustrated in a population-based cohort study of 1195 patients with newly diagnosed or suspected epileptic seizures, 564 of whom had definite epileptic seizures [10]. The proportions of males and females were similar, 25 percent were under the age of 15, and 24 percent were 60 years or older. The majority (62 percent) of epileptic seizures were idiopathic. In the remainder, the cause was vascular disease in 15 percent and tumor in 6 percent. The proportion with an identifiable cause was much higher in older patients; 49 percent were due to vascular disease and 11 percent to tumor. Onset of seizures in late life may be a risk factor for stroke, possibly because covert cerebrovascular disease can often be the mechanism of new onset epilepsy in older patients. This point is illustrated by a study of 4,709 people with idiopathic epilepsy beginning at or after the age of 60 years, but no history of stroke [11]. Subjects were matched to the same number of controls with no history of epilepsy or stroke. In longitudinal follow-up, the epilepsy group had a significantly higher risk of stroke at any time point compared with controls (hazard ratio 2.9, 95% CI 2.45-3.41). This finding suggests that new onset of seizures in older patients should prompt evaluation and treatment for stroke risk factors. Head injury accounts for a relatively small proportion of epilepsy overall. The risk to an individual who suffers head trauma varies widely from minimal risk in people who have a concussive head injury in which the loss of consciousness or amnesia is less than 30 minutes, to at least a 12-fold increased risk over 10 years in people who suffer trauma-induced prolonged amnesia, subdural hematoma, or brain contusion [12]. Antiepileptic drugs prevent seizures in the first week after head injury, but do not prevent the development of epilepsy [13]. (See "Post-traumatic seizures and epilepsy"). Physiological nonepileptic seizures — A number of medical disorders are known to cause physiological NES (show table 1). Hyperthyroidism can cause seizures and can exacerbate seizures in patients with epilepsy. Hypoglycemic seizures are most common in diabetic patients who take excessive amounts of insulin or oral hypoglycemics; islet cell tumors are much less common, but seizures may be the initial presentation. Prodromal symptoms of hypoglycemic seizures include diaphoresis, tachycardia, anxiety, and confusion. Nonketotic hyperglycemia most commonly occurs in elderly diabetics and can cause focal motor seizures. Precipitous falls in serum sodium concentrations can trigger generalized tonic-clonic seizures (see "Generalized seizures" below), usually in association with a prodrome of confusion and depressed level of consciousness. These convulsions are associated with a high risk of mortality and must be treated urgently. (See "Manifestations of hyponatremia and hypernatremia"). Hypocalcemia is a rare cause of seizures and most often occurs in neonates. In adults, hypocalcemia may occur after thyroid or parathyroid surgery or in association with renal failure, hypoparathyroidism, or pancreatitis. Typical prodromic symptoms and signs are mental status changes and tetany. Magnesium levels below 0.8 mEq/L may result in irritability, agitation, confusion, myoclonus, tetany, and convulsions, and may be accompanied by hypocalcemia. (See "Clinical manifestations of hypocalcemia"). Renal failure and uremia are often associated with seizures, particularly myoclonic seizures (see "Generalized seizures" below). Generalized tonic-clonic seizures occur in approximately 10 percent of patients with chronic renal failure, usually late in the course. Seizures may also occur in patients undergoing dialysis as part of the dialysis disequilibrium syndrome; associated symptoms are headache, nausea, muscle cramps, irritability, confusion, and depressed level of consciousness. (See "Seizures in patients undergoing hemodialysis"). Disorders of porphyrin metabolism may cause seizures. Acute intermittent porphyria (AIP) is due to a partial deficiency of porphobilinogen deaminase, which results in excess delta-aminolevulinic acid and porphobilinogen in the urine. Seizures occur in approximately 15 percent of AIP attacks and are usually generalized tonic-clonic seizures, although partial seizures may occur (see "Auras (simple partial seizures)" below and see "Complex partial seizures" below). Other symptoms of AIP include abdominal pain and behavioral changes. (See "Acute intermittent porphyria"). Cerebral anoxia as a complication of cardiac or respiratory arrest, carbon monoxide poisoning, drowning, or anesthetic complication can cause myoclonic and generalized tonic-clonic seizures. Cerebral anoxia due to syncope can result in very brief tonic and/or clonic movements without a prolonged postictal state, which is why syncope frequently results in an evaluation for seizures. (See "Evaluation of the patient with syncope", section on Distinction of syncope from seizures). DIFFERENTIAL DIAGNOSIS — Several conditions must be differentiated from epileptic seizures. REM behavior disorder — REM behavior disorder is a parasomnia that consists of sudden arousals from REM sleep immediately followed by complicated, often aggressive, behaviors for which the patient is amnestic. Diagnosis is clarified by overnight sleep testing (polysomnography). (See "Classification of sleep disorders", section on Parasomnias.) Transient ischemic attack — Transient ischemic attacks (TIAs) may last seconds to minutes. They are generally characterized by "negative" symptoms and signs (such as weakness or visual loss) rather than the "positive" symptoms and signs (eg, jerking movements, stiffening, or visual illusions or hallucinations) that generally accompany seizures. Nevertheless, the postictal state may include lateralizing "negative" symptoms such as weakness; thus, a careful history of possible symptoms that preceded the "negative" symptoms is critical. (See "Differential diagnosis of brain ischemia"). Transient global amnesia — Transient global amnesia (TGA) is a syndrome characterized by the acute onset of severe anterograde amnesia accompanied by retrograde amnesia, without other cognitive or focal neurologic impairment. The amnesia resolves within 24 hours. Most patients are middle aged or older adults. Episodes are usually not recurrent, but some patients have infrequent attacks that recur over several years. The etiology of TGA is uncertain [14]. Most TGA episodes are probably related to vasoconstriction or migraine, but some may be caused by transient ischemia or complex partial seizures. TGA can be associated with small focal abnormalities on diffusion-weighted MRI [15-19], but the significance of these remains unclear. Migraine — Migraine auras such as visual illusions and basilar migraine symptoms, including altered consciousness, can mimic complex partial seizures. Furthermore, the headache that follows complex partial and generalized tonic-clonic seizures is migrainous in quality and duration. (See "Complex partial seizures" below and see "Pathophysiology, clinical manifestations, and diagnosis of migraine in adults"). CLINICAL FEATURES — The diagnostic evaluation of a first seizure begins with the history. An accurate description of the seizure may be difficult to obtain from the patient and witnesses; it is usually necessary to ask pointed questions about the circumstances leading up to the seizure, the ictal behaviors, and the postictal state. It is also worthwhile to inquire specifically whether the patient has had prior seizures, including febrile seizures in infancy, or other episodes that were not evaluated by a physician or that were labeled as something other than seizures. Seizure precipitants or triggers — A key element in the history is whether a particular environmental or physiological precipitant or trigger immediately preceded the seizure. Some patients with epilepsy tend to have seizures under particular conditions, and their first seizure may provide a clue to their so-called seizure trigger. Triggers include (but are not limited to) strong emotions, intense exercise, loud music, and flashing lights. (See "Photic induced seizures" below). These triggers are often experienced immediately before the seizure. Other physiological conditions such as fever, the menstrual period, lack of sleep, and stress can also precipitate seizures, probably by lowering seizure threshold rather than directly causing a seizure. As a result, the temporal relationship to the presenting seizure is often less clear. Triggers may also precipitate physiological NES; a cough, for example, can bring on a syncopal seizure. However, the majority of patients with epilepsy have no identifiable or consistent trigger to their seizures. In addition, triggers are the sole cause of epileptic seizures in only a very small percentage of patients. Photic induced seizures — Photosensitivity has received considerable attention as a seizure trigger. The light stimulation may come from a natural or artificial source, in particular television shows and video games. The most famous incident occurred in relation to a Pokemon cartoon aired in 1997 in Japan in which 685 children (from an estimated 7 million viewers) sought medical attention for neurologic symptoms; most (about 80 percent) were felt to be seizures [20,21]. Three-fourths of the children had not experienced seizures previously. A review of photic-induced seizures made the following epidemiologic observations [21]: Children are more susceptible to photic-induced seizures and photoparoxysmal EEG changes than adults; and photosensitivity may decline in individuals with photic-induced seizures. A tendency for photic-induced seizures may be inherited. Photoconvulsive seizures are usually generalized, but they may be partial. Individuals may be sensitive to certain light triggers but not others. Women appear more susceptible, but males dominate in reports of video game-induced seizures, probably because they play them more. The stimuli that are most likely to induce seizures appear to be identifiable. Guidelines for restricting use of specific signals on television broadcasts exist in Japan and Great Britain, and a working group has developed draft consensus guidelines in the United States [22]. Seizure symptoms and signs — The next step in the history is to identify the symptoms and signs (observed behaviors) that occurred throughout the seizure. Auras (simple partial seizures) — The symptoms that a patient experiences at the beginning of the seizure are referred to as the warning or aura. Auras are seizures that affect enough of the brain to cause symptoms, but not enough to interfere with consciousness. In the seizure classification system established by the International League Against Epilepsy, auras are called simple partial seizures (show table 2); "simple" means that consciousness is not impaired and "partial" means that only part of the cortex is disrupted by the seizure [23]. The symptoms of simple partial seizures vary from one patient to another and depend entirely on where the seizure originates in the brain, that is, the part of the cortex that is disrupted at the onset of the seizure. A seizure that begins in the occipital cortex may result in flashing lights, while a seizure that affects the motor cortex will result in rhythmic jerking movements of the face, arm, or leg on the side of the body opposite to the involved cortex (Jacksonian seizure). Auras that commonly occur in patients with epilepsy are shown in the table (show table 3). These symptoms can also be experienced under other circumstances, but do not typically precede physiological NES. Thus, the occurrence of an aura supports the diagnosis of an epileptic seizure. When a patient's first seizure was not preceded by a simple partial seizure, it is more difficult to distinguish whether it was an epileptic seizure or a NES. Many epileptic patients do not have a warning when their seizures start. Instead, they abruptly lose consciousness, which they may describe as blacking out, when the part of the cortex that controls memory is disrupted by the seizure. However, this process is not specific for epileptic seizures and therefore does not allow differentiation from NES. Complex partial seizures — The classification system for epileptic seizures includes several seizure types that are characterized by an abrupt loss of consciousness: complex partial seizures ("complex" means that consciousness and awareness of the surroundings are lost), absence seizures, and generalized tonic-clonic seizures (also known as convulsions; "tonic" refers to muscle stiffening and "clonic" refers to muscle jerking) (show table 2). Complex partial seizures (previously called temporal lobe seizures or psychomotor seizures) are the most common type of seizure in epileptic adults. During the seizure patients appear to be awake but are not in contact with others in their environment and do not respond normally to instructions or questions. They often seem to stare into space and either remain motionless or engage in repetitive behaviors, called automatisms, such as facial grimacing, gesturing, chewing, lip smacking, snapping fingers, repeating words or phrases, walking, running, or undressing. Patients may become hostile or aggressive if physically restrained during complex partial seizures. Complex partial seizures typically last less than three minutes and may be immediately preceded by a simple partial seizure. Afterward, the patient enters the postictal phase, often characterized by somnolence, confusion, and headache for up to several hours (show table 4). The patient has no memory of what took place during the seizure other than, perhaps, the aura. The behaviors that typify complex partial seizures are not specific for epileptic seizures and may be observed in association with NES. Generalized seizures — In contrast to partial seizures, generalized seizures originate virtually in all the regions of the cortex. Absence seizures and generalized tonic-clonic seizures are types of generalized seizures. Other subtypes of generalized seizures are clonic, myoclonic, tonic, and atonic seizures. Absence seizures usually occur during childhood and typically last between 5 and 10 seconds. They frequently occur in clusters and may take place dozens or even hundreds of times a day. Absence seizures cause sudden staring with impaired consciousness. If an absence seizure lasts for 10 seconds or more, there may also be eye blinking and lip smacking. Absence seizures are discussed in greater detail separately. (See "Epilepsy syndromes in children", section on Absence seizures). A generalized tonic-clonic seizure (also called grand mal seizure, major motor seizure, or convulsion) is the most dramatic type of seizure (show table 5). It begins with an abrupt loss of consciousness, often in association with a scream or shriek. All of the muscles of the arms and legs as well as the chest and back then become stiff. The patient may begin to appear cyanotic during this tonic phase. After approximately one minute, the muscles begin to jerk and twitch for an additional one to two minutes. During this clonic phase the tongue can be bitten, and frothy and bloody sputum may be seen coming out of the mouth. The postictal phase begins once the twitching movements end. The patient is initially in a deep sleep, breathing deeply, and then gradually wakes up, often complaining of a headache. Clonic seizures cause rhythmical jerking muscle contractions that usually involve the arms, neck, and face. Myoclonic seizures consist of sudden, brief muscle contractions that may occur singly or in clusters and that can affect any group of muscles, although typically the arms are affected. Consciousness is usually not impaired. Tonic seizures cause sudden muscle stiffening, often associated with impaired consciousness and falling to the ground. Atonic seizures (also known as drop seizures or drop attacks) produce the opposite effect of tonic seizures — a sudden loss of control of the muscles, particularly of the legs, that results in collapsing to the ground and possible injuries. The behaviors that typify absence seizures and generalized tonic-clonic seizures are not specific for epileptic seizures and may be observed in association with NES. Other aspects of the patient history Medication history — There are a number of medications that have been associated with iatrogenic seizures [8,24]. Partial-onset seizures are less likely to be drug-induced than generalized tonic-clonic seizures. Past medical history — There are a number of risk factors for epileptic seizures that should be addressed, including head injury, stroke, Alzheimer's disease, history of intracranial infection, and alcohol or drug abuse. Family history — A positive family history of seizures is highly suggestive that the patient has epilepsy. In particular, absence seizures and myoclonic seizures may be inherited. Occasionally, a family member does not have seizures but has an abnormal electroencephalogram. Physical and neurologic examination — The physical examination is generally unrevealing in patients with epileptic seizures, but is important when central nervous system infection or hemorrhage are diagnostic possibilities. The neurologic examination should evaluate for lateralizing abnormalities, such as weakness, hyperreflexia, or a positive Babinski sign, that may point to a contralateral structural brain lesion. DIAGNOSTIC STUDIES Laboratory screening — Laboratory evaluations that are appropriate for the evaluation of a first seizure include glucose, calcium, magnesium, hematology studies, renal function tests, and toxicology screens. Tests for porphyria may be considered under appropriate clinical circumstances. Some laboratory abnormalities such as metabolic acidosis and leukocytosis may occur as a result of the seizure; thus, abnormal test results detected immediately after the seizure has occurred should be repeated. Prolactin — Serum prolactin assessment has limited utility as a diagnostic test for epileptic seizures [25]. The serum prolactin concentration may rise shortly after generalized tonic-clonic seizures and some partial seizures. Typically, a level is drawn 10 to 20 minutes after the event and compared with a baseline level drawn six hours later. Criteria for abnormality are not well established; many investigators use twice the baseline level. A systematic review made the following conclusions regarding prolactin as a diagnostic test for epileptic seizures [26]: Pooled sensitivity was higher for generalized tonic-clonic seizures than for partial complex seizures (60 versus 46 percent). An elevated serum prolactin level can be useful in differentiating generalized tonic-clonic and partial complex seizures from psychogenic seizures in adults and older children. The positive predictive value is greater than 93 percent, if the pretest probability is 50 percent or higher. Because of low sensitivity, a normal prolactin level is insufficient to exclude epileptic seizures or support a psychogenic diagnosis. Some studies suggest that prolactin rises after syncope. Prolactin levels cannot be used to differentiate seizure from syncope. Insufficient data preclude conclusions regarding the utility of prolactin levels after simple partial seizures, repetitive seizures, status epilepticus, and in neonates. Lumbar puncture — A lumbar puncture is essential if the clinical presentation is suggestive of an acute infectious process that involves the central nervous system or the patient has a history of cancer that is known to metastasize to the meninges. In other circumstances the test is not likely to be helpful and may be misleading since a prolonged seizure itself can cause cerebrospinal fluid pleocytosis. Lumbar puncture should only be performed after a space occupying brain lesion has been excluded by appropriate neuroimaging studies. Electroencephalography — The electroencephalogram (EEG) is an essential study in the diagnostic evaluation of epileptic seizures. If abnormal, the EEG may substantiate the diagnosis of epileptic seizures and indicate whether a patient may have generalized or partial seizures. Obtaining the EEG in the sleep-deprived state and using provocative measures during the test, such as hyperventilation and intermittent photic stimulation, increase the yield [27]. An abnormal EEG that confirms the clinical diagnosis of epilepsy substantially increases the likelihood that the patient will experience a second seizure over the next two years [28]. However, a normal EEG does not rule out epilepsy, and a positive EEG may be nonspecific. As an example, certain types of EEG abnormalities are seen in patients with migraine headaches or in association with medications. Neuroimaging — A neuroimaging study should be done to exclude a structural brain abnormality if the patient's first seizure was clearly not a physiological NES. Brain magnetic resonance imaging (MRI) is preferred over computed tomography (CT) to identify specific lesions such as cortical dysplasias, infarcts, or tumors. Nevertheless, a brain CT scan is suitable to exclude a mass lesion, hemorrhage, or large stroke under emergency situations or if an MRI is unavailable or contraindicated (eg, in patients with pacemakers, non-compatible aneurysm clips, or severe claustrophobia). The value of neuroimaging in the evaluation of adults with a first seizure was demonstrated in a retrospective review of 148 patients studied within 30 days of the seizure [29]. The cause of seizure was established in 71 patients (48 percent); a structural lesion was identified by CT in 55 (37 percent) and 16 (11 percent) had metabolic seizures. CT findings agreed with the results of neurologic examination in 82 percent of cases. However, structural lesions (including three tumors) were found by CT in 14 patients (15 percent) with nonfocal findings and in 12 (22 percent) patients with generalized EEG abnormalities. In young to middle-aged adults, common MRI findings are mesial temporal sclerosis, sequelae of head injury, congenital anomalies, brain tumors, and vascular lesions. In the elderly, MRIs often reveal strokes, cerebral degeneration, or neoplasms. However, up to 50 percent of patients, regardless of age, have normal neuroimaging studies. The utility of brain MRI in children presenting with a seizure is discussed separately. (See "Clinical and laboratory diagnosis of seizures in infants and children", section on Neuroimaging). WHEN TO START ANTIEPILEPTIC THERAPY — The decision to initiate therapy with antiepileptic drugs is often difficult. This topic is discussed separately. (See "Overview of the management of epilepsy in adults", section Antiepileptic drug therapy). PSYCHOSOCIAL CONSIDERATIONS — Newly diagnosed patients with epilepsy may suffer a number of losses, including loss of independence, employment, insurance, ability to drive, and self-esteem. As the treatment plan is formulated, these psychosocial issues should be explored with patients so that appropriate referrals for additional help and counseling can be initiated. Driving — States vary widely in driver licensing requirements for patients with epilepsy [30]. This topic is discussed in more detail elsewhere. (See "Driving restrictions for patients with seizures and epilepsy"). HOSPITALIZATION — Hospitalization may be required for patients who have a first seizure associated with a prolonged postictal state or incomplete recovery. Other indications for hospitalization include status epilepticus, the presence of a systemic illness that may require treatment, a history of head trauma, or questions regarding compliance. REFERRAL — Many primary care physicians do not feel comfortable with the initial evaluation and management of patients with seizures and refer them to neurologists. All patients in whom the diagnosis is in question should be referred to a neurologist. Other indications for referral include focal findings on the neurologic examination or EEG, or a history suggestive of a focal seizure. Some experts believe that all patients with suspected seizures should be referred to a specialist [31]. CONCLUSIONS — The primary objectives of the medical evaluation of the first seizure are to establish whether it resulted from a correctable systemic process, and if not, whether the patient is at risk for developing further unprovoked seizures. A careful history, physical and neurologic examinations, and laboratory evaluation are usually helpful in achieving these objectives and determining the appropriate therapeutic approach. Referral to a specialist is indicated for most persons suspected of having a seizure, especially if focal features are present on examination or testing. REFERENCES 1. Chang, BS, Lowenstein, DH. Epilepsy. N Engl J Med 2003; 349:1257. 2. Vossler, DG. Nonepileptic seizures of physiologic origin. J Epilepsy 1995; 8:1. 3. Alper, K, Devinsky, O, Perrine, K, et al. Psychiatric classification of nonconversion nonepileptic seizures. Arch Neurol 1995; 52:199. 4. Bortz, JJ. Nonepileptic seizures: issues in differential diagnosis and treatment. CNS Spectrums 1997; 2:20. 5. Berg, AT, Shinnar, S. The risk of seizure recurrence following a first unprovoked seizure: a quantitative review. Neurology 1991; 41:965. 6. Camfield, PR, Camfield, CS, Dooley, JM, et al. Epilepsy after a first unprovoked seizure in childhood. Neurology 1985; 35:1657. 7. Willmore, LJ. Epilepsy emergencies: the first seizure and status epilepticus. Neurology 1998; 51:S34. 8. Schachter, SC. Iatrogenic seizures. Neurol Clin 1998; 16:157. 9. Schold, C, Yarnell, PR, Earnest, MP. Origin of seizures in elderly patients. JAMA 1977; 238:1177. 10. Sander, JW, Hart, YM, Johnson, AL, Shorvon, SD. National General Practice Study of Epilepsy: newly diagnosed epileptic seizures in a general population. Lancet 1990; 336:1267. 11. Cleary, P, Shorvon, S, Tallis, R. Late-onset seizures as a predictor of subsequent stroke. Lancet 2004; 363:1184. 12. Annegers, JF, Hauser, WA, Coan, SP, Rocca, WA. A population-based study of seizures after traumatic brain injuries. N Engl J Med 1998; 338:20. 13. Temkin, NR. Antiepileptogenesis and seizure prevention trials with antiepileptic drugs: meta-analysis of controlled trials. Epilepsia 2001; 42:515. 14. Caplan, LR. Transient global amnesia. In: Handbook of Clinical Neurology, vol 1 (46), Clinical Neuropsychology, Frederiks, JAM (Ed), Vinken, PJ, Bruyn, GW, Klawans (Eds), Elsevier, Amsterdam, 1985. p.205. 15. Woolfenden, AR, O'Brien, MW, Schwartzberg, RE, et al. Diffusion-weighted MRI in transient global amnesia precipitated by cerebral angiography. Stroke 1997; 28:2311. 16. Strupp, M, Bruning, R, Wu, RH, et al. Diffusion-weighted MRI in transient global amnesia: elevated signal intensity in the left mesial temporal lobe in 7 of 10 patients. Ann Neurol 1998; 43:164. 17. Ay, H, Furie, KL, Yamada, K, Koroshetz, WJ. Diffusion-weighted MRI characterizes the ischemic lesion in transient global amnesia. Neurology 1998; 51:901. 18. Sedlaczek, O, Hirsch, JG, Grips, E, et al. Detection of delayed focal MR changes in the lateral hippocampus in transient global amnesia. Neurology 2004; 62:2165. 19. Huber, R, Aschoff, AJ, Ludolph, AC, Riepe, MW. Transient Global Amnesia. Evidence against vascular ischemic etiology from diffusion weighted imaging. J Neurol 2002; 249:1520. 20. Takada, H, Aso, K, Watanabe, K, et al. Epileptic seizures induced by animated cartoon, "Pocket Monster". Epilepsia 1999; 40:997. 21. Fisher, RS, Harding, G, Erba, G, et al. Photic- and pattern-induced seizures: a review for the Epilepsy Foundation of America Working Group. Epilepsia 2005; 46:1426. 22. Harding, G, Wilkins, AJ, Erba, G, et al. Photic- and pattern-induced seizures: expert consensus of the Epilepsy Foundation of America Working Group. Epilepsia 2005; 46:1423. 23. Proposal for revised classification of epilepsies and epileptic syndromes. Commission on Classification and Terminology of the International League Against Epilepsy. Epilepsia 1989; 30:389. 24. Dallos, V, Heathfield, K. Iatrogenic epilepsy due to antidepressant drugs. Br Med J 1969; 4:80. 25. Shukla, G, Bhatia, M, Vivekanandhan, S, et al. Serum prolactin levels for differentiation of nonepileptic versus true seizures: limited utility. Epilepsy Behav 2004; 5:517. 26. Chen, DK, So, YT, Fisher, RS. Use of serum prolactin in diagnosing epileptic seizures: report of the Therapeutics and Technology Assessment Subcommittee of the American Academy of Neurology. Neurology 2005; 65:668. 27. Shinnar, S, Kang, H, Berg, AT, et al. EEG abnormalities in children with a first unprovoked seizure. Epilepsia 1994; 35:471. 28. van Donselaar, CA, Schimsheimer, RJ, Geerts, AT, Declerck, AC. Value of the electroencephalogram in adult patients with untreated idiopathic first seizures. Arch Neurol 1992; 49:231. 29. Ramirez-Lassepas, M, Cipolle, RJ, Morillo, LR, Gumnit, RJ. Value of computed tomographic scan in the evaluation of adult patients after their first seizure. Ann Neurol 1984; 15:536. 30. Krauss, GL, Ampaw, L, Krumholz, A. Individual state driving restrictions for people with epilepsy in the US. Neurology 2001; 57:1780. 31. National Institute for Clinical Excellence (UK). The epilepsies: the diagnosis and management of the epilepsies in adults and children in primary and secondary care. NICE Clinical Guideline 20, Oct. 2004. www.nice.org.uk/page.aspx?o=229249 (Accessed 3/7/05).
-
Cardiac vs Pulmonary Dyspnea - New tool to assess COPD/CHF
Ace844 replied to Buddy's topic in General EMS Discussion
"GAmedic," Do you have any response to this? Anyone lese have anything to add? ACE -
Hello Everyone, Here's an article on this subject. HTH, ACE844 (Withholding Resuscitation: A New Approach to Prehospital End-of-Life Decisions [Article) Feder, Sylvia MA, MICP; Matheny, Roger L. MICP; Loveless, Robert S. Jr PhD, EMT-D; Rea, Thomas D. MD, MPH From King County Medic One and Kent Fire Department, Kent, Washington, and King County Emergency Medical Services and University of Washington, Seattle, Washington. For author affiliations, see end of text. Acknowledgments: The authors thank Dr. Thomas Hearne, Dr. Mickey Eisenberg, Dr. Jack Murray, and John Jerin, King County Emergency Medical Services, for supporting the withholding resuscitation program; Ed Plumlee, King County Medic One, for advocating the program to the local fire chiefs; Linda Becker, King County Emergency Medical Service, for assistance with data acquisition; and Dr. Ned Feder for advice. Grant Support: By the Medic One Foundation and King County Emergency Medical Services. Potential Financial Conflicts of Interest: Grants: S. Feder, R.L. Matheny (Medic One Foundation). Corresponding Author: Sylvia Feder, King County Medic One, 7064 South 220th Street, Kent, WA 98032; e-mail, sylvia_feder@kcfiremed.org. Current author addresses and author contributions are available at www.annals.org . Current Author Addresses: Ms. Feder and Mr. Matheny: King County Medic One, 7064 South 220th Street, Kent, WA 98032. Dr. Loveless: Kent Fire Department, 24611 116 Avenue SE, Kent, WA 98030. Dr. Rea: University of Washington/King County Emergency Medical Services, 999 3rd Avenue, Suite 700, Seattle, WA 98104. Author Contributions: Conception and design: S. Feder, R.L. Matheny. Analysis and interpretation of the data: S. Feder, R.S. Loveless Jr., T.D. Rea. Drafting of the article: S. Feder. Critical revision of the article for important intellectual content: S. Feder, R.L. Matheny, R.S. Loveless Jr., T.D. Rea. Final approval of the article: S. Feder, R.L. Matheny, R.S. Loveless Jr., T.D. Rea. Statistical expertise: R.S. Loveless Jr., T.D. Rea. Obtaining of funding: S. Feder, R.L. Matheny. Administrative, technical, or logistic support: R.L. Matheny. Collection and assembly of data: S. Feder, R.L. Matheny, R.S. Loveless Jr] Abstract Background: Emergency medical services (EMS) personnel often are not permitted to honor requests to withhold resuscitation at the end of life, particularly if there is no written do-not-resuscitate (DNR) order. Objective: To determine whether EMS personnel from agencies implementing new guidelines would be more likely to withhold resuscitation from persons having out-of-hospital cardiac arrests than would personnel from agencies that did not implement the guidelines. Design: Observational study in which 16 of 35 local EMS agencies volunteered to implement new guidelines for withholding resuscitation. Setting: King County, Washington. Patients: 2770 patients with EMS-attended cardiac arrest. Intervention: New guidelines adopted by participating agencies permitted EMS personnel to withhold resuscitation if the patient had a terminal condition and if the patient, family, or caregivers indicated, in writing or verbally, that no resuscitation was desired. Measurements: Proportion of resuscitations withheld in agencies that implemented new guidelines compared with those that did not. Results: Emergency medical services personnel from agencies implementing new guidelines withheld resuscitation in 11.8% of patients (99 of 841 patients) having cardiac arrests, compared with an average of 5.3% (range, 4.2% to 5.9%) of patients (103 of 1929 patients) in 3 historical and contemporary control groups. Honoring verbal requests alone accounted for 53% of withheld resuscitations in the intervention group (52 of 99 patients) compared with an average of 8% (range, 7% to 9%) in the control groups (8 of 103 patients). Limitations: The study was not a randomized, controlled trial; individual agencies chose whether to implement the guidelines. Conclusions: Implementation of new guidelines was associated with an increase in the number of resuscitations withheld by EMS personnel. This increase was primarily due to honoring verbal requests. -------------------------------------------------------------------------------- Emergency medical services (EMS) personnel arrive at a home to find an elderly, emaciated patient in cardiac arrest. Family members state that he had end-stage cancer and did not want life-sustaining measures to be taken. However, there is no formal written do-not-resuscitate (DNR) order. The emergency medical technicians (EMTs) remove the patient from bed, despite the now vehement and emotional objections of the family, and begin resuscitation. This scenario, based on an actual event, shows a predicament sometimes faced by EMS personnel who arrive on the scene and find a patient with a terminal condition who has previously expressed the wish that no resuscitation be attempted. The response of EMS personnel to such patients is often inconsistent and depends on local guidelines, patient presentation, and type of DNR order. As of 1999, 42 states had adopted formal protocols that allowed EMS personnel to withhold resuscitation efforts if the patient had a specific, state-approved prehospital DNR order (1). However, when this is lacking and when resuscitation wishes are conveyed by a different type of directive or by verbal request, EMS personnel usually begin resuscitation. An unwanted resuscitation effort may violate the patient’s right to self-determination, cause suffering and emotional distress for the patient and the family, be troubling for EMS providers, and expend resources unnecessarily. Discussions with providers in our EMS system and our personal experiences underscored the importance of this issue and prompted us to develop new guidelines that gave EMS personnel greater discretion to withhold resuscitation efforts in patients with terminal conditions, even in the absence of state-approved prehospital DNR documentation. The guidelines were implemented by some, but not all, EMS agencies in our county. We hypothesized a priori that EMS personnel from agencies implementing the guidelines would probably withhold resuscitation more often than those from agencies not implementing the guidelines, specifically because the guidelines allowed EMS personnel to honor verbal requests. We also hypothesized that EMS personnel would make decisions consistent with the guidelines and that they would report little difficulty in making these decisions. Methods Study Design, Setting, and Sample This investigation was an observational study of persons having out-of-hospital cardiac arrests in King County, Washington (excluding Seattle), and incorporated historical and contemporary comparison groups (Figure). King County, excluding Seattle, comprises urban, suburban, and rural areas; has a population of approximately 1.2 million persons; and covers 2043 square miles. King County has a 2-tiered EMS response system that has been described previously (2). First-tier firefighter-EMTs are first to arrive on the scene and provide cardiopulmonary resuscitation and defibrillation; second-tier paramedics arrive next and provide advanced life support. -------------------------------------------------------------------------------- [Help with image viewing] [Email Jumpstart To Image] Figure. Study design. “Participating agencies” were defined as those that agreed to implement the 1998 guidelines. “Nonparticipating agencies” were those that did not implement the guidelines. DNR = do not resuscitate; EMS = emergency medical services. -------------------------------------------------------------------------------- The study sample consisted of all cases of out-of-hospital cardiac arrest to which EMS personnel responded, except those for which DNR orders would not typically be pertinent (trauma, suicide, or drug overdose). Although the term cardiac arrest is used by EMS personnel to denote persons who are unconscious and do not have a pulse, there may be cardiac, respiratory, neurologic, or other causes for the patient’s condition. The institutional review board at the University of Washington approved the study. Written informed consent was not required. Verbal consent was obtained from EMS personnel before they were interviewed. Intervention Before 1998, patient care guidelines directed EMS personnel in King County to initiate resuscitation attempts in all persons having cardiac arrest, except those with signs of obvious death (lividity or rigor mortis) or those with a state-approved prehospital DNR directive. Other written directives were occasionally honored, although this was not endorsed. Verbal requests were rarely honored. In 1998, 2 of the present authors, who work as paramedics in King County, proposed new guidelines to address the situation of patients with terminal conditions who did not desire resuscitation and did not have DNR paperwork. These guidelines allowed EMS providers to withhold resuscitation if 1) the patient had a preexisting terminal condition and 2) the patient, family, or caregivers indicated, in writing or verbally, that the patient did not want resuscitation. A terminal condition was defined as one in which death was expected and for which the patient was under a physician’s care. Resuscitation wishes could be communicated through a state-approved prehospital DNR directive, other directive, or verbal request. Although the guidelines were valid for both EMTs and paramedics, we expected that they would be most useful to EMTs, who are first to arrive at the scene. Physician consultation was not necessary to invoke the guidelines. Assistance in developing the guidelines and training program was provided by the King County medical director, local physicians, paramedics, EMS administrators, and a legal consultant (Johnson BR. Liability of EMS personnel for honoring or failing to honor a “Do Not Resuscitate” (DNR) order. Legal opinion paper presented to King County Emergency Medical Services. May 1998). The guidelines and training were offered to all 35 EMS agencies in King County. Participation was voluntary, and each agency’s fire chief made the final decision. Ultimately, 16 agencies chose to participate. Those that did not participate cited legal and risk management concerns. Characteristics of participating and nonparticipating agencies and the populations they serve are shown in Table 1. The 35 agencies have the same countywide medical director, continuing education program, patient care guidelines, and criteria-based dispatch guidelines. -------------------------------------------------------------------------------- [Help with image viewing] [Email Jumpstart To Image] Table 1. Characteristics of Participating and Nonparticipating Agencies -------------------------------------------------------------------------------- Personnel from participating agencies were trained in 1998. Training consisted of a printed manual, written guidelines, a 2-hour lecture and discussion, and a competency quiz. Approximately 900 EMTs and 70 paramedics were trained. Because some agencies participated and some did not, we were able to compare EMS care between these groups. We compared resuscitation decisions for 1-year periods before and after the introduction of the guidelines: preguideline (calendar year 1997, the last full year before guideline implementation) and postguideline (calendar year 1999, the first full year after guideline implementation). Cases occurring in 1998 were excluded because training took place that year. This classification generated 4 groups: preguideline nonparticipating agencies, preguideline participating agencies, postguideline nonparticipating agencies, and postguideline participating agencies. Within these groups, each case of cardiac arrest was classified into 1 of 3 categories: attempted resuscitation, no resuscitation due to signs of irreversible death, or withheld resuscitation. Withheld resuscitation consisted of cases where resuscitation was withheld on the basis of either a written DNR order or verbal request. Data Collection For each EMS response, information was collected from the medical incident report that was completed by EMTs and paramedics. After implementation of the guidelines, 1 of the authors conducted interviews to collect information on cases of withheld resuscitation. Each interview lasted approximately 15 minutes and included 30 questions about the patient, scene, family members, and actions of EMS personnel. The EMS personnel obtained most information from family members (for example, the patient’s history and diagnosis and the reason for the 911 telephone call), although no standard query was used. When possible, the interview took place within 1 day of the telephone call, but there were occasional delays of 1 week or more. Interviews were conducted for 51 of 99 cases of withheld resuscitation. The EMS officer in charge of patient care for each case was interviewed. No persons declined to be interviewed. Statistical Analysis Descriptive statistics summarize the characteristics of the patient sample. A multinomial logistic regression model was used to estimate probabilities for the 3 nominal response categories of attempted resuscitation, no resuscitation, and withheld resuscitation. Independent variables included factors of time (preguideline or postguideline implementation), participating agency (yes or no), patient location (private residence, nursing home, or other), patient sex (male or female), a covariate of patient age in years divided by 100, and an interaction term of time and participating agency. Individual agency identification was not tabulated for each observation or medical incident report; therefore, a random-effects term for agency was not entered into the model. A multiple imputation method using the expectation-maximization algorithm for continuous and categorical data was used for missing data in 89 cases of age and 46 cases of sex. Chi-square tests compared resuscitation wishes (verbal or written) with study group and patient location. Summary statistics were performed with InStat for Macintosh, version 3.0 (GraphPad Software, San Diego, California). Multiple imputations, regression models, and chi-square tests were evaluated by using R, version 2.2.0 (R Core Development Team, 2005) (3). Role of the Funding Source The funding source had no role in the design, analysis, or interpretation of the study or in the decision to submit the manuscript for publication. Results Resuscitation Data During the 2 years of the study (1997 and 1999), 2770 persons had nontraumatic, EMS-attended, out-of-hospital cardiac arrests. The characteristics of the patient sample are shown in Table 2. -------------------------------------------------------------------------------- [Help with image viewing] [Email Jumpstart To Image] Table 2. Characteristics of Patients Who Had Cardiac Arrest according to Resuscitation Effort -------------------------------------------------------------------------------- Across all agencies, resuscitation was withheld in 5.9% (78 of 1333) of patients having cardiac arrests before guideline implementation and in 8.6% (124 of 1437) of patients having cardiac arrests after guideline implementation. Specifically, resuscitations were withheld from 11.8% of patients in the postguideline participating group compared with 5.8%, 5.9%, and 4.2% in the preguideline nonparticipating group, preguideline participating group, and postguideline nonparticipating group, respectively (Table 3). -------------------------------------------------------------------------------- [Help with image viewing] [Email Jumpstart To Image] Table 3. Type of Resuscitation Effort according to Study Group -------------------------------------------------------------------------------- The analysis using multinomial logistic regression indicated that the probability of withholding resuscitation varied by time period (preguideline or postguideline) and agency participation (P < 0.001 for the agency participation × time interaction term for withheld resuscitation vs. attempted resuscitation and withheld resuscitation vs. no resuscitation). On the basis of the results of the model, the postguideline participating group more than doubled its probability of withholding resuscitation compared with the other study groups after controlling for patient age, sex, and location. Table 4 shows the means by which resuscitation wishes were conveyed (state directive, other directive, or verbal request). The difference between the postguideline participating group and each of the other 3 groups is largely due to the honoring of verbal requests, which made up 53% (52 of 99) of withheld resuscitations in the former group and only 8% (8 of 103) in the latter groups (P < 0.001). Thus, when responding to verbal requests, the postguideline participating group increased its probability of withholding resuscitation more than 6-fold compared with other study groups. -------------------------------------------------------------------------------- [Help with image viewing] [Email Jumpstart To Image] Table 4. Type of Request To Withhold Resuscitation according to Study Group -------------------------------------------------------------------------------- In the postguideline participating group, there was an association between type of directive and patient location. Patients residing in nursing care facilities were more likely to have a written directive, whereas those residing in private homes were more likely to have family members verbally express resuscitation wishes (P = 0.003) (Table 5). -------------------------------------------------------------------------------- [Help with image viewing] [Email Jumpstart To Image] Table 5. Type of Request To Withhold Resuscitation in the Intervention Group according to Patient Location -------------------------------------------------------------------------------- Interviews with EMS Personnel Emergency medical services personnel in the postguideline participating group were interviewed for 51 of 99 cases of withheld resuscitation. The characteristics of patients seen by interviewed and noninterviewed EMS personnel were similar (interviewed vs. noninterviewed: mean age, 77.1 years vs. 80.3 years; male sex, 41% vs. 43%; private home, 61% vs. 58%; verbal request to withhold resuscitation, 52% vs. 54%). Both conditions necessary to invoke the guidelines (terminal condition and request to withhold resuscitation) were documented on the medical incident report in all cases of withheld resuscitation in the postguideline participating group. Interviews with EMS providers supported the assertions made on the medical incident report; in no case was there a discrepancy, although the interviews often provided more detail. Of the 51 patients, 18 (35%) had terminal cancer; 32 (63%) had another terminal condition, such as renal failure; and 1 had an unknown terminal condition with hospice in attendance. Seven patients were said to be in a persistent vegetative state. Forty-five of the patients (88%) were in cardiac arrest on EMS arrival; the remainder progressed to cardiac arrest within minutes of EMS arrival. Communication of resuscitation wishes by either written or verbal means was provided in all cases—by family in 34 cases (67%) and by staff or caregivers in 17 cases (33%). The EMS personnel who were interviewed indicated that they had little difficulty in making decisions about withholding resuscitation on the basis of the guidelines. Forty-six (90%) rated the decision to withhold resuscitation as “easy,” 4 rated it as “moderate,” and none found the decision “difficult.” Emergency medical services personnel indicated that the guidelines affected their decision to withhold resuscitation. Thirty-two of 51 (63%) said they would have initiated or continued a resuscitation effort in a similar situation before guideline implementation, particularly in cases in which there was a verbal request only (81%). The EMS personnel who were interviewed reported that there were no objections, complaints, or disagreements among family members or other persons on the scene when resuscitation efforts were withheld, nor was any such objection noted on the medical incident report of any case. Paramedic units were initially dispatched in 48 of 51 cases (94%) about which EMS personnel were interviewed. The EMTs at the scene canceled paramedic units 23 times (48%) while the units were en route. In the remaining 25 cases, paramedics arrived and assisted the family but did not override the decision of the EMTs and begin resuscitation. Persons who called 911 gave reasons in 41 of 51 cases. In 23 residences, the most common reasons were “didn’t know what else to do” (26%), wanted confirmation of death (22%), and needed medical assistance (48%). The latter category included patients whose dying process included coughing or vomiting blood, seizures, agonal respirations, or severe dyspnea. In all 6 nursing home cases, staff called 911 because they had not determined the patient’s DNR status. The remaining 12 cases took place in adult family homes and reflected a mix of the aforementioned reasons. In addition, 3 people called because they thought 911 notification was required by law. Discussion In this observational study, 16 of 35 EMS agencies in King County implemented guidelines permitting EMS personnel to withhold resuscitation from persons having out-of-hospital cardiac arrests. The implementation of these guidelines was associated with a statistically significant increase in the number of resuscitations withheld by EMS personnel in participating agencies compared with those in nonparticipating agencies or historical experience. This increase was primarily due to honoring verbal requests. Medical incident reports and interviews indicated that EMS personnel appropriately followed the guidelines for withholding resuscitation. Previous studies (4-6) indicate that EMS assistance is requested during some cases of cardiac arrest even when resuscitation is not wanted. In our study, a telephone call to 911 was made for various reasons, including the need for medical assistance or for confirmation of death. Means of conveying resuscitation wishes range from a prehospital DNR order to a verbal request. In metropolitan Toronto, Canada, 70% of DNR requests were verbal (6) compared with approximately 50% in this study. In the absence of formal written DNR documentation, EMS personnel typically attempt resuscitation. In our study, most EMS personnel who were interviewed stated that they would have initiated resuscitation in a similar situation before the implementation of the new guidelines. This finding is supported by a national survey of more than 1500 EMTs in which 89% stated they would honor a state-approved advance directive, 10% stated they would honor a verbal report of an advance directive, and only 4% said they would honor an “unofficial” document (7). There is no mention of honoring a verbal request alone. Emergency medical services personnel who are prevented from honoring a request to withhold resuscitation may act in ways that are in no one’s interest. The authors of the Toronto study wrote, “In several cases resuscitation was initiated despite the repeated objections of caregivers. In the face of these objections, the paramedics sometimes had to move the patient to the ambulance, out of sight of the caregivers, to begin resuscitation, knowing full well that this delay would render the attempt futile” (6). The issue of unwanted resuscitation has sometimes been addressed by educating families not to call 911 (6). This approach may be unrealistic; families overwhelmed by the realities of death may call 911 regardless of instructions. Interviews with EMS personnel indicated that almost 80% of calls from private homes were initiated because family members sought medical assistance or “didn’t know what else to do.” Telling families not to call 911 may also deprive them of the compassionate support that EMS personnel can provide. The act of calling 911 for help should not lead to an unwanted resuscitation. Another approach to limit unwanted resuscitation is a statewide prehospital DNR directive. However, a survey conducted 6 years after implementation of the directive in our state found that 60% of physicians did not know that this directive was required in the prehospital setting (8). In the intervention group of our study, only 4% of patients in whom resuscitation was withheld had the state directive, whereas 43% had a different type of written advance directive. Permitting EMS personnel to honor various written directives will not address every situation; more than 50% of the resuscitation requests in our study were conveyed by verbal statements alone. Such measures as instructing families not to call 911 or implementing a statewide prehospital DNR plan will prevent some unwanted resuscitation attempts. However, these measures probably will not eliminate the problem. The guidelines we described provide a well-received and locally implementable approach that addresses the issue at the level of the EMS provider; it is at this level that decisions must be made if the other strategies have failed. Since the completion of the study in 1999, the withholding resuscitation program has continued in King County and is currently being expanded to include agencies that did not initially participate. We are not aware of other EMS programs in the United States that permit EMS personnel to withhold resuscitation by using similar guidelines. This investigation has several limitations. The 16 participating agencies were not selected randomly but voluntarily implemented the guidelines. The remaining agencies declined to participate, citing legal and risk management concerns. Consequently, the increased proportion of withheld resuscitations after guideline implementation may be specific to agencies that actively use the guidelines and perhaps cannot be generalized to other agencies. The self-selecting nature of the study groups can lead to unrecognized clustering factors that may mask or lend false significance to some effects (9). A second limitation is that not all EMS providers who withheld resuscitation were interviewed. However, noninterviewed personnel, according to characteristics collected from the medical incident reports, were similar to those who were interviewed. A third limitation is that bias may have been introduced because data obtained from the medical incident reports were collected by the authors, who were not blinded to the study group. A fourth limitation is the possibility of inaccurate statements given by EMS personnel in the medical incident reports and interviews. Finally, although the guidelines for withholding resuscitation were well received by EMS personnel, the impact on family members and caregivers was not well studied. The EMS personnel who were interviewed did not report any objections by family or caregivers when resuscitations were withheld; in fact, there were anecdotes relaying the gratitude of family members for the support provided by EMS personnel. However, this was not investigated systematically. Interviews with family members and caregivers are the next step in evaluating this program. In conclusion, the implementation of guidelines for withholding resuscitation was associated with an increase in the proportion of withheld resuscitations, primarily due to honoring verbal requests. The EMS personnel appropriately followed the guidelines and indicated that they had little difficulty making these decisions. Of importance, the program is continuing in the original agencies, and implementation has begun in those agencies that did not initially participate. The success of this program suggests that it could be incorporated into a community-wide approach to expected natural deaths occurring outside of a hospital. We believe that such a program, when carried out appropriately within a supportive EMS infrastructure, can improve care at the end of life by preserving patient autonomy and diminishing suffering. We feel these features benefit the patients, their families, the EMS providers, and the community as a whole. See also: Print Editors’ Notes 635 Editorial comment 692 Web-Only Conversion of figure and tables into slides Context Emergency medical services (EMS) personnel attending out-of-hospital cardiac arrests sometimes attempt to resuscitate patients who do not want life-sustaining measures. Contribution This study involving 2770 patients with EMS-attended cardiac arrests assessed outcomes of guidelines that permitted personnel to withhold resuscitation in patients with terminal illnesses when the patients or caregivers indicated, in writing or verbally, that they did not want resuscitation. Sixteen of 35 EMS agencies in King County, Washington, implemented the guidelines. Implementation and control agencies withheld resuscitation in approximately 11.8% and 5.3% of cardiac arrests, respectively. The difference was primarily due to honoring verbal requests. Cautions In this observational study, self-selected agencies volunteered to implement guidelines. —The Editors References 1. Sabatino CP. Survey of state EMS-DNR laws and protocols. J Law Med Ethics. 1999;27:297-315, 294. [PMID: 11067612] SFX Bibliographic Links Library Holdings [Context Link] 2. Rea TD, Eisenberg MS, Becker LJ, Murray JA, Hearne T. Temporal trends in sudden cardiac arrest: a 25-year emergency medical services perspective. Circulation. 2003;107:2780-5. [PMID: 12756155] Ovid Full Text Bibliographic Links Library Holdings [Context Link] 3. R Development Core Team. R: a language and environment for statistical computing. R Foundation for Statistical Computing. 2005. Accessed at www.R-project.org on 1 October 2005. [Context Link] 4. Becker LJ, Yeargin K, Rea TD, Owens M, Eisenberg MS. Resuscitation of residents with do not resuscitate orders in long-term care facilities. Prehosp Emerg Care. 2003;7:303-6. [PMID: 12879377] SFX Bibliographic Links Library Holdings [Context Link] 5. Dull SM, Graves JR, Larsen MP, Cummins RO. Expected death and unwanted resuscitation in the prehospital setting. Ann Emerg Med. 1994;23:997-1002. [PMID: 8185118] SFX Bibliographic Links Library Holdings [Context Link] 6. Guru V, Verbeek PR, Morrison LJ. Response of paramedics to terminally ill patients with cardiac arrest: an ethical dilemma. CMAJ. 1999;161:1251-4. [PMID: 10584085] Ovid Full Text Bibliographic Links Library Holdings [Context Link] 7. Marco CA, Schears RM. Prehospital resuscitation practices: a survey of prehospital providers. J Emerg Med. 2003;24:101-6. [PMID: 12554050] SFX Bibliographic Links Library Holdings [Context Link] 8. Silveira MJ, Buell RA, Deyo RA. Prehospital DNR orders: what do physicians in Washington know? J Am Geriatr Soc. 2003;51:1435-8. [PMID: 14511164] SFX Bibliographic Links Library Holdings [Context Link] 9. Localio AR, Berlin JA, Ten Have TR, Kimmel SE. Adjustments for center in multicenter studies: an overview. Ann Intern Med. 2001;135:112-23. [PMID: 11453711] Ovid Full Text Bibliographic Links Library Holdings [Context Link] Fast Tracking Annals will consider manuscripts of high quality for expedited review and early publication (Fast Track) if they have findings that are likely to affect practice immediately and if they are judged valid. We give priority to fast-tracking large clinical trials with clinical outcomes. Authors wishing to fast track their articles should send Dr. Cynthia Mulrow (cynthiam@acponline.org) an electronic version of their manuscript along with a request and justification for expedited review.
-
(Annals of Emergency Medicine Volume 47 @ Issue 6 , June 2006, Pages 515-524 doi:10.1016/j.annemergmed.2006.01.012 Copyright © 2006 American College of Emergency Physicians Published by Mosby, Inc. Emergency medical service/airway/review article Economic Value of Out-of-Hospital Emergency Care: A Structured Literature Review Presented at the International Interdisciplinary Conference on Emergencies, June 2005, Montreal, Quebec, Canada. E. Brooke Lerner PhDa, , , Ronald F. Maio DO, MSb, Herbert G. Garrison MD, MPHc, Daniel W. Spaite MDd and Graham Nichol MDe aDepartment of Emergency Medicine and Department of Community and Preventive Medicine, University of Rochester, Rochester, NY bDepartment of Emergency Medicine, University of Michigan, Ann Arbor, MI cDepartment of Emergency Medicine, East Carolina University, Greenville, NC dDepartment of Emergency Medicine, College of Medicine, University of Arizona, Tucson, AZ eUniversity of Washington–Harborview Center for Prehospital Emergency Care and Emergency Services, Harborview Medical Center, University of Washington, Seattle, WA. Received 9 November 2005; revised 5 January 2006; accepted 6 January 2006. Available online 24 March 2006.) Study objective The evaluation of the impact of out-of-hospital emergency care is a relatively new research focus. As such, there is a compelling need to determine how finite health care resources should be used in this setting. The objective of this study is to conduct a structured review of published economic evaluations of out-of-hospital emergency care to assess its economic value. Methods A structured literature search and structured review of articles pertaining to the economic value of out-of-hospital care was performed. The bibliographic database MEDLINE was searched for pertinent English-language articles published between 1966 and 2003. The search used the medical subject headings “emergency medical services” and “emergency medical technician” and was limited to the subheading “economics” and crossed with the medical subject heading “economics.” The titles generated by this search were systematically reviewed and limited by topic. Abstracts from the identified titles were reviewed to select a final set of pertinent articles. These articles were further limited based on explicit inclusion and exclusion criteria. Authors used a previously published structured evaluation tool to review the final set of identified articles for quality and content. Results The initial MEDLINE search identified 3,533 citations. From this set, 535 potentially relevant abstracts were reviewed. From the abstract review, 46 articles were identified, along with an additional 14 from searching the secondary references. Of these 60 articles, 32 met the review inclusion criteria and were subjected to a full structured review. These studies predominantly addressed the cost of cardiac arrest (n=13, 41%), major trauma (n=8, 25%), and emergency medical services treatment in general (n=8, 25%). Only 14 studies considered the costs and consequences of competing alternatives. Of these, 2 were cost-benefit and 12 were cost-effectiveness evaluations. Two of the 14 studies met all 10 criteria for high-quality economic evaluation, whereas 2 others met none. Conclusion There is a paucity of out-of-hospital care literature that addresses cost and economic value. The extant literature is limited in scope, poor in quality, and evaluates small subsets of out-of-hospital emergency care costs. Favorable cost-effectiveness has not been firmly established for most aspects of out-of-hospital emergency care. Introduction Background Although few would argue the need for out-of-hospital emergency care within a community, which services and resources should be provided and rapidity of response are topics of considerable debate.1, 2, 3, 4 and 5 As new technologies and skills emerge, decisions about which services a community should provide become even more difficult. Recent studies such as the Ontario Prehospital Life Support project have evaluated the effectiveness of several out-of-hospital interventions.6, 7 and 8 However, determining which out-of-hospital interventions to use within a community is more than merely a matter of efficacy and effectiveness. To properly make this determination, cost and cost-effectiveness must also be considered. Out-of-hospital emergency medical services (EMS) is a relatively young field. Therefore, the level of research has been nascent.5 and 9 The natural evolution of research can be thought of as a continuum from laboratory to efficacy to effectiveness research. Economic evaluations done in a variety of settings are at the end of this continuum.6 Once effectiveness has been established and there are at least 2 competing alternatives, economic evaluations are used to determine which alternative is more desirable from a cost perspective. There are myriad out-of-hospital care activities that need to be evaluated from a cost perspective to assist communities in determining how their finite resources should be used. Interest in out-of-hospital care is high, as evidenced by the recent creation of an out-of-hospital subcommittee for the committee on the Future of Emergency Care in the United States at the Institute of Medicine.10 Simultaneously, health care costs are increasing at alarming rates.11 In the case of out-of-hospital emergency care, a significant proportion of this cost is often paid by taxpayers, who subsidize the out-of-hospital emergency care system to maintain its availability. For example, in Los Angeles the Reason Public Policy Institute found that the cost per patient transport was $476, and, because of a low rate of fee collection, there was a $12 per-capita tax subsidy required to maintain the system.12 The current study was conducted to determine the evidence available on the cost of out-of-hospital emergency care in general, as well as various out-of-hospital interventions. The objective was to systematically review published economic evaluations of out-of-hospital emergency care in order to assess its economic value. Materials and methods Data Sources A structured literature search and a structured review of articles pertaining to the economic value of out-of-hospital emergency care were performed. The initial phase consisted of a search of the bibliographic database MEDLINE to identify relevant English-language articles published between 1966 and 2003. An initial set of references was developed by searching for the medical subject headings “emergency medical services” and “emergency medical technician.” These medical subject headings were limited to the subheading “economics.” A second search was conducted by not limiting the medical subject headings and cross-referencing them by using the “and” command, with a third medical subject heading “economics.” A secondary review was then performed by searching the reference sections of all identified articles for additional relevant articles. Study Selection A single investigator (E.B.L.) reviewed this initial set of reference titles to identify those articles that potentially addressed the cost or economic value of out-of-hospital care. Titles for which it was obvious that out-of-hospital emergency care was not addressed were excluded. We created a working definition of “out-of-hospital emergency care.” It was defined as acute, unscheduled health care delivered outside the hospital by a system that deploys health resources in response to calls to a public safety answering point. Any title that failed to indicate that a cost or economic evaluation was conducted was excluded. However, to ensure that no relevant articles were missed, any title that could possibly contain a cost or economic evaluation of out-of-hospital emergency care was included for further review. The abstracts of the selected reference titles were obtained and reviewed by 2 investigators (E.B.L., R.F.M.). Abstracts that indicated that the manuscript might discuss some aspect of the evaluation of out-of-hospital care from a cost or economic perspective were included for further analysis. Again, any questionable abstracts were retained for further review. The full articles for the selected abstracts were retrieved and reviewed. A single author (E.B.L.) reviewed all of the manuscripts and selected those that met the study definition of out-of-hospital emergency care. Editorials, non–peer-reviewed reports, and review articles were excluded, as well as strictly methods articles. Several investigators (E.B.L., R.F.M., H.G.G., D.W.S.) read the remaining articles and categorized them into 3 groups by using a consensus process. Each categorization was discussed until there was unanimous agreement on all decisions. The categories were those that did any type of cost analysis, those that mentioned the word “cost” but failed to analyze costs, and those that had no mention of cost. The reference sections of all articles that were reviewed were hand searched for additional relevant articles. Data Extraction All articles that reported economic analyses of out-of-hospital EMS were evaluated using a previously published checklist for assessing economic evaluations developed by Drummond et al.13 Before the checklist was applied, the articles were classified by whether they were full or partial economic evaluations and by the type of economic evaluation (Figure 1). Drummond defined economic evaluations as full evaluations if they compared the costs and consequences of 2 or more competing alternatives.13 (27K) Figure 1. Method of categorizing full economic evaluations and partial evaluations. By permission of Oxford University Press. Adapted from Methods for the Economic Evaluation of Healthcare Programmes, 2nd edition.13 Four types of full economic evaluations were considered: cost-benefit, cost-effectiveness, cost utility, and cost minimization. In a cost-benefit analysis, consequences are measured in dollars. In a cost-effectiveness analysis, consequences are measured in natural units such as the amount of disability or health care resources consumed. In a cost-utility analysis, consequences are measured in quality-adjusted life years (some authors consider a cost-utility analysis to be a cost-effectiveness study with a more comprehensive outcome that accounts for both the quality and quantity of life).14 A cost-minimization analysis compares 2 competing alternatives with equivalent outcomes to determine which results in the lowest cost. Cost minimization can also be considered a form of cost-effectiveness analysis in which the effectiveness of the competing alternatives is equivalent. Once the type of evaluation was identified, all references were assessed with a checklist for assessing economic evaluations described by Drummond et al13 (Table 1). This checklist was selected because it is comprehensive and widely accepted in the field of health economics. Table 1. Structured review of articles using the check list for assessing economic evaluations published by Drummond et al.13⁎ First Author (Year) Type of Analysis Well-defined Question Comprehensive Description of Competing Alternatives Effectiveness Established Important and Relevant Costs and Consequences for Each Alternative Costs and Consequences Measured Accurately in Appropriate Physical Units Costs and Consequences Valued Credibly Costs and Consequences Adjusted for Differential Timing Incremental Analysis of Costs and Consequences of Alternatives Performed Allowance Made for Uncertainty in the Estimates of Costs and Consequences Presentation and Discussion of Study Results Include All Issues of Concern to Users Riediger (1990)15 Cost benefit Cretin (1977)16 Cost benefit De Wing (2000)17 Cost minimization Brazier (1996)18 Cost minimization Turner (2000)19 Cost minimization Nichol (2003)20 Cost utility Nichol (1996)21 Cost utility Nichol (1998)22 Cost utility Jermyn (2000)23 Cost-effectiveness Ornato (1988)24 Cost-effectiveness Urban (1981)25 Cost-effectiveness Hallstrom (1981)26 Cost-effectiveness Forrer (2002)27 Cost-effectiveness Valenzuela (1990)28 Cost-effectiveness Suchard (1999)29 Cost description Rosemurgy (1993)30 Cost description Daberkow (1977)31 Cost description Pascarelli (1978)32 Cost description Kriegsman (1998)33 Cost description Altintas (1999)34 Cost description Snooks (1996)35 Cost description Jackobsson (1987)36 Cost description Kurola (2002)37 Cost outcome description Gearhart (1997)38 Cost outcome description Bur (2001)39 Cost outcome description Hauswald (1997)40 Cost outcome description Brady (1996)41 Cost analysis Lammers (1995)42 Cost analysis Fischer (2000)43 Cost analysis Lechleuthner (1994)44 Cost analysis Bruhn (1993)45 Cost analysis Nicholl (1994)46 Cost analysis ⁎ Checkmarks indicate that the criteria were present. Results Data Synthesis The initial MEDLINE search created a reference set of 3,533 references (Figure 2). From this set, 535 references were selected for abstract review, and of these, 46 were selected for a complete manuscript review. Searching the reference sections of selected manuscripts identified an additional 14 references. Of these 60 articles, 32 met the review inclusion criteria and were subjected to a full structured review by several authors (E.B.L., R.F.M., D.W.S., H.G.G.) (Table 1). (41K) Figure 2. Article analysis. Of the 32 studies identified, only 14 were full economic evaluations.15, 16, 17, 18, 19, 20, 21, 22, 23, 24, 25, 26, 27 and 28 Of these evaluations, 2 were cost-benefit analyses.15 and 16 The remaining 12 studies were some form of cost-effectiveness study. Three were cost-minimization analyses.17, 18 and 19 Three studies used quality-adjusted life years as the outcome.20, 21 and 22 The remaining 6 studies were traditional cost-effectiveness studies that described the outcome in natural units, primarily cost per life saved.23, 24, 25, 26, 27 and 28 Eighteen of the 32 studies were identified as partial economic evaluations. Eight were cost descriptions: they examined only the cost of 1 alternative, there was no comparison, or the consequences or outcomes were not considered.29, 30, 31, 32, 33, 34, 35 and 36 Four were cost-outcome descriptions, meaning that they examined only 1 alternative but considered the costs and the consequences of that alternative.37, 38, 39 and 40 Six were cost-analyses because they examined the cost of 2 alternative treatments but not the consequences.41, 42, 43, 44, 45 and 46 Using the checklist for economic evaluations, the investigators evaluated how well the articles met the checklist criteria (Table 1). Two of the 14 full economic evaluation studies met none of the 10 checklist criteria,15 and 17 whereas 2 of the studies met all of the checklist criteria.19 and 21 The others covered various amounts of the checklist criteria. Among the 32 studies, 13 (41%) addressed interventions for cardiac arrest, 8 (25%) investigated major trauma (1 study addressed both cardiac arrest and major trauma), and 8 (25%) investigated the general cost of an EMS system. Of the 14 full economic evaluations, 9 (64%) addressed cardiac arrest and 3 (21%) studied major trauma. There was 1 partial analysis that specifically addressed pediatric patients. Among the 14 full economic evaluations, 4 reported that they calculated costs from the societal perspective. The remaining 10 studies did not clearly state the perspective they were using. However, it appears that 4 used a societal perspective and 6 used the EMS perspective in calculating costs. In terms of comparing the outcomes of the 14 full economic evaluations, only 9 studies reported which calendar year’s dollars were used to calculate costs, thus making difficult the comparison of the other 5 studies to each other or other economic evaluations of interventions (Table 2). Table 2. Results of the full economic evaluation studies. Article by First Author Type of Evaluation Perspective EMS Cost Factors Year in Which Dollars Are Expressed Conclusion Riediger (1990)15 Cost benefit Not stated, appears societal Few details given Not stated EMS saves $44.3 million per year to the community, and the cost-effective ratio for EMS is 5.3. Cretin (1977)16 Cost benefit Not stated, appears societal Attendant salary and benefits, equipment, support personnel, maintenance, and other costs Not stated It costs between $3,141 and $6,100 per added year of life for a mobile coronary care unit, depending on how the cost is discounted. De Wing (2000)17 Cost minimization Not stated, appears agency Used charges by the agency Not stated There is a 7- to 8-fold increase in charges for helicopter transport compared to ground transport. There is a potential $500,000 saving over 2 years if ground transport used instead. Brazier (1996)18 Cost minimization Not stated, appears societal Aircraft, pilots, medical personnel, maintenance, landing facilities, and ambulance control staff involved in deployment Fiscal year 1991–1992 The incremental cost of helicopter transport was $1.97 million annually. Turner (2000)19 Cost minimization Not stated, appears societal Consumable equipment, cost per minute on call Fiscal year 1997–1998 No statistically significant difference in cost between the 2 groups. Nichol (2003)20 Cost utility Societal Defibrillator (cost depreciated), nontraditional responder training (including instructor costs), nontraditional responder retraining (including cost of drills) 2003 Nontraditional responders had an incremental cost of a median $56,700 per QALY gained. Nichol (1996)21 Cost utility Societal 911 Service, ambulance communication center, ambulance bases, vehicles, equipment, wages, benefits, and education 1991 Incremental cost of adding more EMS providers in a single-tier EMS system was $368,000 per QALY. In a 2-tier system in which more BLS is added, the cost is $53,000 per QALY if they respond in fire department pump vehicles or $159,000 per QALY if respond in ambulances. Changing from a 1-tier system to a 2-tier system was $40,000 per QALY in a pump vehicle or $94,000 per QALY in an ambulance. Nichol (1998)22 Cost utility Societal AED annual training, AED maintenance, police defibrillator 1996 Incremental cost of PAD by lay providers was $44,000 per QALY or $27,200 per QALY when PAD was provided by a police officer. Jermyn (2000)23 Cost-effectiveness Not stated, appears agency Defibrillators, ancillary equipment, biomedical services for preventive maintenance on defibrillators, routine nonwarranty work, training equipment, trainer/provider certification, call review by medical control, attrition of providers and trainers 1996 Canadian dollars Cost per life saved in an urban area was $6,776; $49,274 for a rural area. Ornato (1988)24 Cost-effectiveness Not stated, appears agency EMT training, ambulance, radio equipment, medical equipment (eg, stretcher, oxygen, first aid supplies), defibrillator, UHF radio for telemetry, and drug box Not stated Total initial training personnel and equipment cost per life saved from ventricular defibrillation was $7,687 for a basic EMT, $2,125 for an EMT-D, and $2,289 for a paramedic. Urban (1981)25 Cost-effectiveness Societal Paramedic training (depreciated during 5 years), equipment, vehicle (depreciated during 2–5 years), salaries, benefits, relief pay, supplies, support services, equipment, maintenance, dispatching, management, vehicle operating and maintenance 1978 The marginal cost per life saved was between $6,521 and $42,358 for a suburban paramedic program in regard to cardiac arrest. Hallstrom (1981)26 Cost-effectiveness Not stated, appears agency Could not be determined Not stated For an EMT-only system, the cost-effectiveness (calculated as cost in thousands of dollars divided by percentage of cardiac arrest patients discharged alive) was 52.6 for an EMT defibrillation program and 25.6 when a paramedic fly car was added. Forrer (2002)27 Cost-effectiveness Not stated, appears societal Defibrillator training costs included the instructor stipend and overtime for officer training time. Equipment costs included the cost of the defibrillator, battery, pads, and other miscellaneous equipment. Miscellaneous costs included equipment maintenance costs. 1999 The cost per life saved as a result of decreasing time to first shock with a PAD program was between $23,542 and $70,342, with a cost per year of life saved of between $1,582 and $16,060. Valenzuela (1990)28 Cost-effectiveness Not stated, appears agency Cost included 4 items: personnel costs, which were the dollar difference between ALS and ILS personnel salary and benefits; training costs, including the cost to train an ALS provider per year; equipment costs, including the cost of maintaining monitor defibrillator units on all vehicles; maintaining response time, which was achieved by adding 1 more unit. 1989 The cost per year of life saved was $8,886. EMS, Emergency medical services; QALY, quality adjusted life year; AED, automated external defibrillator; PAD, public access defibrillator; EMT, emergency medicine technician; EMT-D, emergency medicine technician-defibrillator; ALS, advanced life support; ILS, intermediate life support. Among the 10 analyses in which cost per life saved could be compared, we observed large variations in estimation of cost per life saved, reflecting differences in the comprehensiveness of the costs included, as well as the nature of the interventions considered and their estimated effectiveness. These studies evaluated a range of EMS interventions for cardiac arrest. Using the available data, we next estimated cost per life saved expressed in 2005 dollars (Table 3). For studies that did not state the year of data collection, we assumed that costs were calculated in the year of publication. Insufficient data were available on cost per life saved to include any of the other identified studies in this analysis. Table 3. Summary of published economic evaluations of EMS interventions for cardiac arrest, with all results adjusted for inflation. First Author (Year) Intervention Comparator Perspective Costs Included Incremental Cost: Effectiveness, $/Life Saved Nichol (1996)21 Reducing response time for cardiac arrest Existing EMS agency Society EMS and initial hospital costs only; not ICD or ambulatory costs In all-ALS system by adding ALS providers: $2,627,00 In BLS+ALS system by adding defibrillator-capable first responders in: fire vehicles: $383,500 EMS vehicles: $1,134,400 Changing from an all-ALS system to a BLS+ALS system by adding defibrillator-capable first responders in: fire vehicles: $286,900 EMS vehicles: $678,2000 Ornato (1988)24 Treatment by range of EMT Existing EMS agency Agency Training costs only EMT: $12,900 Defibrillation-capable EMT: $3,600 Paramedic: $3,800 Urban (1981)25 Advanced cardiac life support Existing EMS agency Societal EMS and initial hospital costs only $91,900 Valenzuela (1990)28 Advanced cardiac life support Existing EMS agency Agency Training, equipment, and on-time costs $181,000 Jackobsson (1987)36 EMTs Existing EMS agency Societal EMS and hospital only $2,800 Jermyn (2000)23 First-responder defibrillation Existing EMS agency Agency Training and consumables but not capital equipment In urban setting: $7,800 In rural setting: $57,400 Hallstrom (1981)26 Defibrillation EMTs without defibrillation Agency EMS costs assumed By EMTs: $48,100 By EMTs with paramedic backup: $80,900 Forrer (2002)27 Defibrillation Existing EMS agency Agency Training and equipment but not medical oversight or quality assurance By police: $84,000 Nichol (1998)22 Defibrillation Standard EMS agency Society EMS and hospital but not ambulatory or ICD costs By police: $107,000 By lay responders: $190,000 Nichol (2003)20 Defibrillation Standard EMS agency Society EMS, hospital, ICD, and ambulatory costs By security guards in casinos: $183,000 BLS, Basic life support; ICD, implantable cardioverter-defibrillator. Discussion Only 32 studies that were economic analyses of out-of-hospital care could be identified by rigorous evaluation of the past 38 years of peer-reviewed health care literature. In general, economic evaluations are most useful when there is evidence that an intervention is effective. Only a handful of out-of-hospital interventions have been proven unequivocally to be effective.5, 47 and 48 The paucity of science about the effectiveness and cost of out-of-hospital EMS dramatically compromises the ability of communities to optimize the use of limited health care resources. This comprehensive review yielded only 14 full economic evaluations. The majority of studies were partial economic evaluations that failed to compare competing alternatives or to consider both the costs and consequences of the interventions. Although partial evaluations have some utility, the optimal purpose of an economic evaluation is to assist in making choices about the use of community resources.13 Therefore, accurate assessment of the economics of EMS care requires full economic evaluations. Only 8 of the 14 full economic evaluations met a majority of the criteria for a high-quality analysis. Therefore, there are even fewer studies in the medical literature of sufficient quality to truly assist community decisionmakers. These findings support our initial hypothesis about the near absence of high-quality out-of-hospital-care economic analyses. EMS cost analyses must follow guidelines for the standardization of economic analysis, such as those suggested by the Panel on Cost-effectiveness in Health and Medicine,14 but there is also a need for a conceptual framework specific to EMS to assist EMS researchers in designing and reporting economic evaluations. The existing full economic evaluations primarily analyzed cardiac arrest and major trauma. These 2 areas account for only a small percentage of adults transported within the EMS system and an even smaller proportion of pediatric patients transported.49 Therefore, the existing economic literature applies to only a small proportion of patients treated by EMS providers. To meaningfully assist communities in determining how their limited resources should best be used, the scope of economic analyses in out-of-hospital emergency care needs to be expanded and the quality improved. How much a society is willing to pay for EMS will vary between communities and depends, in part, on total funds available. Therefore, different communities are likely to be willing to pay for out-of-hospital care according to their available resources and the competing alternatives, making it difficult to create a universal definition of what incremental cost should be considered a decisionmaking criterion for different EMS systems. It also means that the cost of readiness may be higher in some communities compared to others because of their system demands (eg, minimum response times). Finally, because of the heterogeneous nature of out-of-hospital emergency care agencies, the effectiveness of a given intervention can be highly variable between agencies.50 This factor makes it difficult to determine overall cost-effectiveness for a given intervention across all out-of-hospital emergency care agency types. To our knowledge, this is the first comprehensive literature search on the economic evaluation of out-of-hospital emergency care. It included an objective assessment of the literature conducted by multiple authors using previously validated criteria for identifying high-quality analyses. The state of the literature on economic evaluation of EMS is poor. Recommendations Future evaluations of the economics of out-of-hospital emergency care interventions should apply rigorous methods to define the population under consideration, the intervention, what it is being compared to, the perspective of the study, the effects of the intervention and its alternatives, and their costs.14 Limitations This study was a comprehensive literature search that applied a priori qualitative criteria. However, it had several limitations. The existing literature is sparse. It is available for only a few interventions. The methods used were variable between studies, complicating our ability to pool the results meaningfully. Comparisons were even further complicated by the variability between studies in terms of time and place. Our search was limited in that we restricted it to only published English-language literature because of the expense of translation and our inability to identify unpublished studies. Further, because an expert panel conducted this review, it is limited by the biases inherent in a review by only a handful of individuals. However, because consensus required unanimity among all the reviewers and explicit review criteria were used, these biases were limited. Conclusion In conclusion, there is a paucity of literature that addresses the economics of out-of-hospital emergency care. The existing literature is of poor quality, limited to a narrow scope, and does not reflect the broad array of care provided within EMS systems. A conceptual framework for evaluating costs and consequences of care delivered in the out-of-hospital setting is needed. Future studies should evaluate the costs and consequences of out-of-hospital interventions by using high-quality methods. Only with the development of the methodologies for this purpose will the much-needed understanding of how to optimize EMS care be possible. References 1 I.G. Stiell, G.A. Wells and D.W. Spaite et al., The Ontario Prehospital Advanced Life Support (OPALS) Study, part II: rationale and methodology for trauma and respiratory distress patients: OPALS Study Group, Ann Emerg Med 34 (1999), pp. 256–262. SummaryPlus | Full Text + Links | PDF (77 K) 2 I.G. Stiell, G.A. Wells and D.W. Spaite et al., The Ontario Prehospital Advanced Life Support (OPALS) Study rationale and methodology for cardiac arrest patients, Ann Emerg Med 32 (1998), pp. 180–190. SummaryPlus | Full Text + Links | PDF (80 K) 3 D.C. Cone, E.B. Lerner and D.M. Yealy, Prehospital care research the basics, Prehosp Emerg Care 6 (2002), pp. S2–S8. Abstract-MEDLINE | Abstract-EMBASE 4 J.S. Seidel, D. Henderson and S. Tittle et al., Priorities for research in emergency medical services for children results of a consensus conference, Ann Emerg Med 33 (1999), pp. 206–210. SummaryPlus | Full Text + Links | PDF (57 K) 5 M.R. Sayre, L.J. White and L.H. Brown et al., National EMS research agenda, Prehosp Emerg Care 6 (2002), pp. S1–S43. Abstract-EMBASE 6 I.G. Stiell, G.A. Wells and B.J. Field et al., Improved out-of-hospital cardiac arrest survival through the inexpensive optimization of an existing defibrillation program: OPALS Study Phase II: Ontario Prehospital Advanced Life Support, JAMA 281 (1999), pp. 1175–1181. Abstract-EMBASE | Abstract-MEDLINE | Full Text via CrossRef 7 I.G. Stiell, G.A. Wells and V.J. DeMaio et al., Modifiable factors associated with improved cardiac arrest survival in a multicenter basic life support/defibrillation system: OPALS Study Phase I results: Ontario Prehospital Advanced Life Support, Ann Emerg Med 33 (1999), pp. 44–50. SummaryPlus | Full Text + Links | PDF (82 K) 8 I.G. Stiell, G.A. Wells and P.C. Hebert et al., Association of drug therapy with survival in cardiac arrest limited role of advanced cardiac life support drugs, Acad Emerg Med 2 (1995), pp. 264–273. Abstract-MEDLINE 9 D.W. Spaite, E.A. Criss and T.D. Valenzuela et al., Emergency medical service systems research problems of the past, challenges of the future, Ann Emerg Med 26 (1995), pp. 146–152. SummaryPlus | Full Text + Links | PDF (717 K) 10 Institute of Medicine. The future of emergency care: subcommittee on prehospital emergency medical services [institute of Medicine Web site]. Available at: http://www.iom.edu/project.asp?id=20238. Accessed October 10, 2005. 11 F. Sloan, Valuing Health Care, Cambridge University Press, Cambridge, UK (1995). 12 Eggers W. Competitive government for a competitive Los Angeles [Reason Public Policy Institute Web site]. Available at: http://www.rppi.org/es182.html. Accessed October 10, 2005. 13 M. Drummond, B. O’Brien and G. Stoddart et al., Methods for the Economic Evaluation of Health Care Programmes (2nd ed.), Oxford University Press, Oxford, UK (1997). 14 M.R. Gold, J.E. Siegel and L.B. Russell et al., Cost-effectiveness in Health and Medicine, Oxford University Press, Oxford, UK (1996). 15 G. Riediger and T. Fleischmann-Sperber, Efficiency and cost-effectiveness of advanced EMS in West Germany, Am J Emerg Med 8 (1990), pp. 76–80. Abstract 16 S. Cretin, Cost-benefit of treatment and prevention of myocardial infarction, Health Serv Res 12 (1977), pp. 174–189. Abstract-MEDLINE | Abstract-EMBASE 17 M.D. De Wing, T. Curry and E. Stephenson et al., Cost-effective use of helicopters for the transportation of patients with burn injuries, J Burn Care Rehabil 21 (2000), pp. 535–540. Abstract-EMBASE | Abstract-MEDLINE 18 J. Brazier, J. Nicholl and H. Snooks, The cost and effectiveness of the London helicopter emergency medical service, J Health Serv Res Policy 1 (1996), pp. 232–237. Abstract-MEDLINE 19 J. Turner, J. Nicholl and L. Webber et al., A randomised controlled trial of prehospital intravenous fluid replacement therapy in serious trauma, Health Tech Assess 4 (2000), pp. 1–57. Abstract-MEDLINE 20 G. Nichol, T. Valenzuela and D. Roe et al., Cost-effectiveness of defibrillation by targeted responders in public settings, Circulation 108 (2003), pp. 697–703. Abstract-EMBASE | Abstract-Elsevier BIOBASE | Abstract-MEDLINE | Full Text via CrossRef 21 G. Nichol, A. Laupacis and I.G. Stiell et al., Cost-effectiveness analysis of potential improvements to emergency medical services for victims of out-of-hospital cardiac arrest, Ann Emerg Med 27 (1996), pp. 711–720. SummaryPlus | Full Text + Links | PDF (996 K) 22 G. Nichol, A.P. Hallstrom and J.P. Ornato et al., Potential cost-effectiveness of public access defibrillation in the United States, Circulation 97 (1998), pp. 1315–1320. Abstract-MEDLINE | Abstract-EMBASE 23 B.D. Jermyn, Cost-effectiveness analysis of a rural/urban first-responder defibrillation program, Prehosp Emerg Care 4 (2000), pp. 43–47. Abstract-MEDLINE | Abstract-EMBASE 24 J.P. Ornato, E.J. Craren and E.R. Gonzalez et al., Cost-effectiveness of defibrillation by emergency medical technicians, Am J Emerg Med 6 (1988), pp. 108–112. Abstract 25 N. Urban, L. Bergner and M.S. Eisenberg, The costs of a suburban paramedic program in reducing deaths due to cardiac arrest, Med Care 19 (1981), pp. 379–392. Abstract-MEDLINE | Abstract-EMBASE 26 A. Hallstrom, M.S. Eisenberg and L. Bergner, Modeling the effectiveness and cost-effectiveness of an emergency service system, Soc Sci Med 15C (1981), pp. 13–17. Abstract 27 C.S. Forrer, R.A. Swor and R.E. Jackson et al., Estimated cost effectiveness of a police automated external defibrillator program in a suburban community 7 years’ experience, Resuscitation 52 (2002), pp. 23–29. Abstract 28 T.D. Valenzuela, E.A. Criss and D. Spaite et al., Cost-effectiveness analysis of paramedic emergency medical services in the treatment of prehospital cardiopulmonary arrest, Ann Emerg Med 19 (1990), pp. 1407–1411. Abstract 29 J.R. Suchard, F.R. Fenton and R.D. Powers, Medicare expenditures on unsuccessful out-of-hospital resuscitations, J Emerg Med 17 (1999), pp. 801–805. SummaryPlus | Full Text + Links | PDF (74 K) 30 A.S. Rosemurgy, P.A. Norris and S.M. Olson et al., Prehospital traumatic cardiac arrest the cost of futility, J Trauma Injury Infect Crit Care 35 (1993), pp. 468–473. 31 S.G. Daberkow, Location and cost of ambulances serving a rural area, Health Serv Res 12 (1977), pp. 299–311. Abstract-EMBASE 32 E.F. Pascarelli and I.B. Katz, Planning and developing a prehospital mobile intensive care system in an urban setting, Am J Public Health 68 (1978), pp. 389–393. Abstract-MEDLINE | Abstract-EMBASE 33 W. Kriegsman and W. Anthes, The financial impact of alcohol-related emergencies on a rural EMS system, Alaska Med 40 (1998), pp. 7–11. Abstract-MEDLINE 34 K.H. Altintas, N. Bilir and M. Tuleylioglu, Costing of an ambulance system in a developing country, Turkey costs of Ankara Emergency Aid and Rescue Services’ (EARS) ambulance system, Eur J Emerg Med 6 (1999), pp. 355–362. 35 H.A. Snooks, J.P. Nicholl and J.E. Brazier et al., The costs and benefits of helicopter emergency ambulance services in England and Wales, J Public Health Med 18 (1996), pp. 67–77. Abstract-EMBASE | Abstract-MEDLINE 36 J. Jakobsson, O. Nyquist and N. Rehnqvist et al., Cost of a saved life following out-of-hospital cardiac arrest resuscitated by specially trained ambulance personnel, Acta Anaesthesiol Scand 31 (1987), pp. 426–429. Abstract-MEDLINE 37 J. Kurola, M. Wangel and A. Uusaro et al., Paramedic helicopter emergency service in rural Finland do benefits justify the cost?, Acta Anaesthesiol Scand 46 (2002), pp. 779–784. Abstract-MEDLINE | Abstract-EMBASE | Full Text via CrossRef 38 P.A. Gearhart, R. Wuerz and A.R. Localio, Cost-effectiveness analysis of helicopter EMS for trauma patients, Ann Emerg Med 30 (1997), pp. 500–506. SummaryPlus | Full Text + Links | PDF (647 K) 39 A. Bur, H. Kittler and F. Sterz et al., Effects of bystander first aid, defibrillation and advanced life support on neurologic outcome and hospital costs in patients after ventricular fibrillation cardiac arrest, Intensive Care Med 27 (2001), pp. 1474–1480. Abstract-EMBASE | Abstract-MEDLINE | Full Text via CrossRef 40 M. Hauswald and E. Yeoh, Designing a prehospital system for a developing country estimated cost and benefits, Am J Emerg Med 15 (1997), pp. 600–603. SummaryPlus | Full Text + Links | PDF (447 K) 41 W.J. Brady Jr, H. Hennes and A. Wolf et al., Pattern of basic life support ambulance use in an urban pediatric population, Am J Emerg Med 14 (1996), pp. 250–253. SummaryPlus | Full Text + Links | PDF (501 K) 42 R.L. Lammers, B.A. Roth and T. Utecht, Comparison of ambulance dispatch protocols for nontraumatic abdominal pain, Ann Emerg Med 26 (1995), pp. 579–589. SummaryPlus | Full Text + Links | PDF (944 K) 43 A.J. Fischer, P. O’Halloran and P. Littlejohns et al., Ambulance economics, J Public Health Med 22 (2000), pp. 413–421. Abstract-MEDLINE | Abstract-EMBASE | Full Text via CrossRef 44 A. Lechleuthner, W. Koestler and M. Voigt et al., Helicopters as part of a regional EMS system a cost-effectiveness analysis for three EMS regions in Germany, Eur J Emerg Med 1 (1994), pp. 159–166. Abstract-MEDLINE 45 J.D. Bruhn, K.A. Williams and R. Aghababian, True costs of air medical vs. ground ambulance systems, Air Med J 12 (1993), pp. 262–268. Abstract 46 J.P. Nicholl, N.R. Beeby and J.E. Brazier, A comparison of the costs and performance of an emergency helicopter and land ambulances in a rural area, Injury 25 (1994), pp. 145–153. Abstract 47 M.R. Sayre, L.J. White and L.H. Brown et al., The national EMS research strategic plan, Prehosp Emerg Care 9 (2005), pp. 255–266. Abstract-EMBASE | Abstract-MEDLINE | Full Text via CrossRef 48 T.R. Delbridge, B. Bailey and J.L. Chew Jr et al., EMS Agenda for the future: where we are: where we want to be, Prehosp Emerg Care 2 (1998), pp. 1–12. Abstract-MEDLINE 49 R.F. Maio, H.G. Garrison and D.W. Spaite et al., Emergency Medical Services Outcomes Project I (EMSOP I) prioritizing conditions for outcomes research, Ann Emerg Med 33 (1999), pp. 423–432. SummaryPlus | Full Text + Links | PDF (84 K) 50 M.S. Eisenberg, B.T. Horwood and R.O. Cummins et al., Cardiac arrest and resuscitation a tale of 29 cities, Ann Emerg Med 19 (1990), pp. 179–186. Abstract Supervising editor: Donald M. Yealy, MD Funding and support: This work was funded by the National Highway Traffic Safety Administration through Cooperative Agreement DTNH22-98-H-05117 awarded to the National Association of State EMS Directors. The sponsor played no role in the design and conduct of the study; collection, management, analysis, and interpretation of the data; or preparation, review, or approval of the manuscript. Reprints not available from the authors. Address for correspondence: E. Brooke Lerner, PhD, Department of Emergency Medicine, University of Rochester, 601 Elmwood Ave, Box 655, Rochester, NY 14642; 585-273-1468, fax 585-473-3516
-
(Annals of Emergency medicine Volume 47 @ Issue 6, Pages 532-541 (June 2006) Out-of-Hospital Endotracheal Intubation: Where Are We? Henry E. Wang, MD, MPH, Donald M. Yealy, MD Received 27 October 2005; received in revised form 9 January 2006; accepted 11 January 2006 published online 27 February 2006.) While remaining prominent in paramedic care and beneficial to some patients, out-of-hospital endotracheal intubation has not clearly improved survival or reduced morbidity from critical illness or injury when studied more broadly. Recent studies identify equivocal or unfavorable clinical effects, adverse events and errors, interaction with other important resuscitation interventions, and challenges in providing and maintaining procedural skill. We provide an overview of current data evaluating the overall effectiveness, safety, and feasibility of paramedic out-of-hospital endotracheal intubation. These studies highlight our limited understanding of out-of-hospital endotracheal intubation and the need for new strategies to improve airway support in the out-of-hospital setting. Introduction Paramedic out-of-hospital endotracheal intubation originated in the 1970s from efforts to improve outcomes from cardiac arrest and major trauma.1, 2, 3, 4, 5 At that time, the best available methods for paramedic out-of-hospital airway management and ventilation were bag-valve-mask ventilation and the esophageal obturator airway.6, 7, 8, 9 Bag-valve-mask performance was perceived to be inadequate, and esophageal obturator airway use resulted in many complications, including inadequate or delayed ventilation, aspiration, pharyngeal and esophageal injury, gastric rupture, tracheal occlusion, and inadvertent tracheal intubation.6, 8, 10, 11, 12, 13, 14, 15 Out-of-hospital endotracheal intubation offered an alternative method to optimize care, promising superior airway protection, efficient ventilation, and a route to deliver endobronchial medications.16 Endotracheal intubation was also the standard for in-hospital resuscitation, classified as a “definitely helpful” intervention by then-current Advanced Cardiac Life Support guidelines.17 Several authors reported groundbreaking efforts to implement out-of-hospital endotracheal intubation in Boston, Columbus, San Diego, and Pittsburgh.1, 2, 3, 4 SEE EDITORIAL, P 542. Despite its accepted role in clinical practice for more than 25 years, a growing body of literature suggests that out-of-hospital endotracheal intubation is not achieving its intended overarching goals. In selected cases, the intervention may cause harm. In this article, we provide an overview of recent data evaluating the effectiveness, safety, and feasibility of paramedic out-of-hospital endotracheal intubation. Is Out-of-Hospital Endotracheal Intubation Effective? The fundamental test of a medical intervention is whether it improves the outcome of the targeted patients.18 In this light, the overarching goal of out-of-hospital endotracheal intubation is to reduce mortality and morbidity for those in need of airway support. Several investigators have evaluated survival and neurologic outcome after out-of-hospital endotracheal intubation19, 20, 21, 22, 23, 24, 25, 26, 27, 28, 29, 30, 31, 32, 33 (Table). These studies largely involve retrospective analyses of predominantly injured patients. Although 2 studies identified increased survival from out-of-hospital endotracheal intubation, the remaining efforts found either decreased or no effect on survival. No studies have identified improved neurologic outcome from out-of-hospital endotracheal intubation. Table. Studies evaluating survival or neurologic outcome after out-of-hospital endotracheal intubation.⁎ Study Design Primary Population Primary Comparison (Group Sizes) Primary Finding Bochicchio et al, 200329 Prospective observational; single trauma center (Baltimore); univariable/stratified Severe TBI; ETI in field or ED OOH-ETI (78) vs ED-ETI (113) Higher mortality (OR 2.1; 95% CI 0.9–5.0)†‡ in OOH-ETI group Bulger et al, 200519 Retrospective; single trauma center (Seattle); multivariable adjusted Severe TBI; RSI or ETI in field OOH-RSI (775) vs OOH-ETI (302) Higher mortality (OR 1.6; 95% CI 1.0–2.4) and poorer neurologic outcome (1.7; 1.2–2.6) in OOH-ETI group Christensen and Hoyer, 200330 Retrospective; single mobile emergency unit with anesthetist (Denmark) All trauma; ETI in field with and without drugs OOH-ETI with (62) vs without (12) drugs Higher mortality (OR 15.2; 95% CI 1.9–673.2)† for OOH-ETI without drugs Cooper et al, 200131 Retrospective; National Pediatric Trauma Registry; univariable Severe pediatric TBI OOH-ETI (479) vs OOH-BVM (99) No difference in mortality (OR 1.0; 95% CI 0.6-1.6)† Davis et al, 200320 Prospective interventional series, historical controls; countywide (San Diego); multivariable adjusted Severe TBI; RSI in field vs non-ETI historical controls OOH-RSI (209) vs non-OOH-ETI (627) Higher mortality (OR 1.6; 95% CI 1.1–2.2) and poorer neurologic outcome (1.6; 1.2–2.3) in OOH-RSI group Davis et al, 200521 Retrospective; countywide trauma registry (San Diego) multivariable adjusted Severe TBI; ETI in field or ED OOH-ETI (2,665) vs ED-ETI (2,220) Higher mortality (OR 2.1; 95% CI 1.8–2.5)† in OOH-ETI group DiRusso et al, 200532 Retrospective; National Pediatric Trauma Registry; multivariable adjusted All pediatric trauma OOH-ETI (1,928) vs non–trauma center ETI (1,647), trauma center ETI (1,874) and non-ETI (44,739) Higher mortality for OOH-ETI vs non–trauma center ETI (OR 3.2; 95% CI 2.7–3.7)†§; vs trauma center ETI (4.1; 3.5–4.8)†§; vs non-ETI (142.0; 119.6–168.5)†§ Poorer neurologic outcome for OOH-ETI vs non–trauma center or trauma center ETI∥ Gausche et al, 200022 Prospective controlled (pseudorandomized) interventional trial; countywide (Los Angeles) Pediatrics; ETI or BVM in field OOH-ETI/BVM (420) vs OOH-BVM (410) No difference in mortality (OR 0.8; 95% CI 0.6–1.1) or neurologic outcome (0.9; 0.6–1.2) Lockey et al, 200123 Retrospective; single air medical service (Great Britain); descriptive All trauma; ETI in field without drugs Mortality of OOH-ETI without drugs (486) Low (0.2%) survival Murray et al, 200024 Retrospective; countywide trauma registry (Los Angeles); multivariable matched/adjusted Severe TBI OOH-ETI (57) vs non-OOH-ETI (57) Higher mortality (OR 4.2; 95% CI 2.1–8.9) in OOH-ETI group Sloane et al, 200025 Retrospective; single trauma center (San Diego); univariable Severe TBI; RSI in field or ED OOH-RSI (47) vs ED-RSI (267) No difference in mortality (OR 0.6; 95% CI 0.1–2.6)† or neurologic outcome (1.1; 0.3–3.8)† Stockinger et al, 200426 Retrospective; single trauma center (New Orleans); univariable/stratified All trauma; ETI or BVM in field OOH-ETI (316) vs OOH-BVM (217) Higher mortality (OR 18.0; 95% CI 11.2–29.1)† in OOH-ETI group Suominen et al, 200033 Retrospective; single trauma center (Finland); univariable Severe pediatric TBI OOH-ETI (24) vs non–trauma center ETI (13) vs trauma center ETI (22) Lower mortality for OOH-ETI vs non–trauma center ETI (OR 0.1; 95% CI 0.002–1.1)†‡; no difference vs trauma center ETI (3.7; 0.9–15.8)† Wang et al, 200427 Retrospective; statewide trauma registry (Pennsylvania); multivariable and propensity-score adjusted Severe TBI; ETI in field or ED OOH-ETI (1,797) vs ED-ETI (2,301) Higher mortality (OR 4.0, 95% CI 3.2–4.9), poorer neurologic outcome (1.6; 1.2–2.3), and poorer functional outcome (severe impairment 1.9; 1.3–2.5) in OOH-ETI group Winchell and Hoyt, 199728 Retrospective; countywide trauma registry (San Diego); univariable/stratified Blunt trauma, GCS score ≤8 OOH-ETI (527) vs non–OOH-ETI (565) Lower mortality (OR 0.6; 95% CI 0.5–0.8)† in OOH-ETI group; no difference in neurologic outcome (1.4; 1.0–1.9)† BVM, Bag-valve-mask ventilation; ETI, endotracheal intubation; GCS, Glasgow Coma Scale; OOH, out-of-hospital; RSI, rapid sequence intubation; TBI, traumatic brain injury. ⁎ Only the primary findings (survival and neurologic outcome) are summarized; results of other outcomes and subgroup analyses are not presented. † Odds ratio calculated from published results. ‡ Author stated significant at P<.05. § Calculated univariable odds ratios; multivariable adjusted figures not published. ∥ Odds ratios could not be calculated from published results. Of these out-of-hospital endotracheal intubation studies, the most notable effort is by Gausche et al.22 In this prospective trial, 830 critically ill pediatric out-of-hospital patients in Los Angeles County received either bag-valve-mask ventilation or bag-valve-mask followed by out-of-hospital endotracheal intubation. The authors found that an airway strategy incorporating out-of-hospital endotracheal intubation offered no survival or neurologic benefit over bag-valve-mask ventilation alone. Although limited by its patient population (primarily pediatric patients in a large urban location), this seminal effort represents the largest prospective, controlled evaluation of out-of-hospital airway management interventions. The San Diego Rapid Sequence Intubation Trial tested the large-scale implementation of rapid sequence intubation performed by ground-based paramedic units.20 In this outcomes analysis, 209 traumatic brain injury patients receiving out-of-hospital rapid sequence intubation (neuromuscular blockade–assisted endotracheal intubation) were matched to 627 historical nonintubated controls, facilitating a comparison of out-of-hospital rapid sequence intubation with the alternative of no out-of-hospital endotracheal intubation at all. The authors observed higher mortality in patients receiving out-of-hospital rapid sequence intubation (odds ratio 1.6; 95% confidence interval [CI] 1.1 to 2.2). This study is notable because of its large-scale evaluation of one of the most advanced airway management techniques. In the evaluation of this series of studies (listed in the Table and discussed above), several important observations arise about their methods, designs, and limitations. Most of these efforts used retrospective designs involving a single-center or countywide trauma registry and included primarily injured or head-injured patients.19, 20, 21, 23, 24, 25, 26, 27, 28, 29, 30, 31, 32, 33 The Gausche study is the only effort using a prospective, controlled design in medical (nontrauma) cases.22 The studies noted in the Table evaluated survival to hospital discharge only; they did not study long-term outcomes and often inferred neurologic outcome from discharge destination (ie, discharge to home, rehabilitation center, psychiatric facility, or jail = “good neurologic outcome”; discharge to nursing home or other extended care facility = “poor” neurologic outcome). None used formal neurologic or functional measures such as the Glasgow Outcome Scale or the Functional Independence Measure.34, 35 Risk adjustment is important in retrospective analyses because many confounding factors can lead to adverse outcome, not just the manner of airway management.36 However, only some authors used multivariable adjustment to account for these variations.19, 20, 21, 24, 27, 32 Several studies using univariable analysis (including the 2 efforts identifying a survival benefit for out-of-hospital endotracheal intubation) may not have adequately accounted for confounders.28, 33 For example, Winchell and Hoyt28 analyzed 1,098 head-injured patients and found that out-of-hospital endotracheal intubation increased survival by 21%, but this effort did not adjust for severity of injury or illness. Suominen et al33 evaluated 59 pediatric traumatic brain injury patients and found that out-of-hospital endotracheal intubation resulted in a 34% increase in survival over patients intubated in the emergency department (ED) of a referring hospital; however, this small effort similarly did not adjust for severity of injury or illness. The comparison (exposure) groups in these studies differ, each posing a different scientific question. For example, the Davis et al,20 Murray et al,24 and Winchell and Hoyt28 studies used controls that did not receive out-of-hospital endotracheal intubation; we do not know whether these individuals later received ED endotracheal intubation or no endotracheal intubation at all. In our analysis of intubated traumatic brain injury patients in Pennsylvania, we excluded patients not receiving endotracheal intubation in either the out-of-hospital or ED setting, hence facilitating a comparison of endotracheal intubation during the acute out-of-hospital or ED phases only.27 In contrast, the Bulger et al19 study compared out-of-hospital conventional and rapid sequence endotracheal intubation techniques, excluding patients not intubated in the out-of-hospital setting. This latter study compares different out-of-hospital endotracheal intubation techniques, not out-of-hospital endotracheal intubation versus non-out-of-hospital endotracheal intubation. In summary, few studies have demonstrated benefit from out-of-hospital endotracheal intubation.28, 33 Most showed an adverse or no effect on outcome.20, 21, 22, 24, 26, 27, 29, 31, 32 These observations contradict the assumption that aggressive airway intervention is associated with improved resuscitation outcomes. Larger studies with prospective designs may be instrumental in revealing an undetected benefit. However, an important alternate reaction is to recognize that multiple studies arrived at similar conclusions and identified substantial effect sizes, despite their differing populations, disease groups, designs, and limitations. If not directly causative, out-of-hospital endotracheal intubation may have close parallel relationships with factors leading to adverse outcome. A logical direction is to better identify the underlying relationships. Is Out-of-Hospital Endotracheal Intubation Safe? Therapeutic “safety” refers to freedom from accidental injury during the course of medical care.37 Adverse events and errors may contribute to the poor outcomes associated with out-of-hospital endotracheal intubation. This relationship is plausible, given that out-of-hospital endotracheal intubation is a complex procedure with many potential pitfalls, including key errors (eg, unrecognized esophageal tube placement) that can result in morbidity or death. Errors may be more likely in the uncontrolled out-of-hospital environment than in other settings.38 Several recent efforts highlight the underrecognition of out-of-hospital endotracheal intubation errors. Katz and Falk39 evaluated 108 paramedic endotracheal intubation patients arriving at a regional trauma center in Florida. The authors used a systematic physician approach to confirm proper tube placement on ED arrival, including the selected use of direct revisualization. The authors found that more than 25% of the endotracheal tubes were misplaced, two thirds of these in the esophagus. The authors partially attributed the results to noncompliance with out-of-hospital protocols requiring placement confirmation using carbon dioxide detection. Jemmett et al40 conducted a similar study of 109 paramedic endotracheal intubation patients in Maine (an emergency medical services [EMS] system with no carbon dioxide detection protocol) and found a similar tube misplacement rate of 12%. Jones et al41 reported a lower (5.8%) tube misplacement rate for 208 paramedic endotracheal intubation in Indianapolis, but this study occurred in a region serviced primarily by a single EMS agency with close medical oversight. Dunford et al42 examined a subset from the San Diego Rapid Sequence Intubation Trial, finding that accidental oxygen desaturation (SaO2 <90%) occurred in 31 of 54 (57%) patients and marked bradycardia (pulse rate <50 beats/min) in 6 of 54 (19%) patients. Moreover, in 84% of these adverse events, the paramedic described the intubation effort as “easy.” Ehrlich et al43 compared field (out-of-hospital), referring hospital and trauma center endotracheal intubation of pediatric trauma patients. Complications (esophageal intubation, mainstem intubation, aspiration, barotrauma, incorrect tube size, tube dislodgment) occurred in two thirds of the 59 out-of-hospital endotracheal intubations. Self-reporting methods are often used to identify medical errors in the in-hospital setting.37 In the Gausche et al22 study, of 186 initially successful endotracheal intubations, paramedics reported unrecognized esophageal intubation in 2%, tube dislodgment in 14%, and mainstem intubation in 18%. In a prospective multicenter effort involving 45 EMS services, we demonstrated the feasibility of using anonymous, structured, closed-form, self-reporting forms to identify out-of-hospital endotracheal intubation errors.44 Of 1,953 endotracheal intubations, tube misplacement (esophageal, delayed recognition or unrecognized, or dislodgment) was reported in 61 (3.1%) intubations, multiple endotracheal intubation attempts (4 or more laryngoscopies) occurred in 62 (3.2%) intubations, and endotracheal intubation efforts failed in 359 (18.5%) intubations. More than 22% of patients experienced 1 or more of these errors or complications. Although these data are limited by self-reporting biases and a moderate return rate (68%), they still identify worrisome “best case” error rate estimates; that is, true error rates are likely to be higher, not lower, than reported with this design. These data highlight our incomplete awareness of and limited ability to identify out-of-hospital endotracheal intubation errors. For example, the Katz and Falk study highlighted that out-of-hospital endotracheal intubation is prone to detection bias; errors go undetected unless systematically identified.39 Cases series describing tracheal and pulmonary injury from out-of-hospital endotracheal intubation indicate that many errors are identified only through invasive tests or autopsy.45, 46 Dunford et al42 showed that during the course of routine care, rescuers are often unaware of adverse events, even when equipped with the most advanced monitoring techniques. Although none of these efforts formally linked out-of-hospital endotracheal intubation errors to outcome, these events have plausible connections with patient outcome. These studies highlight our underrecognition and incomplete understanding of the range of errors occurring during out-of-hospital endotracheal intubation. Does Out-of-Hospital Endotracheal Intubation Affect Other Aspects of Care? Current paramedic textbooks portray endotracheal intubation as a procedure to be completed independent of other patient care tasks.47 However, other interventions often occur concurrently with endotracheal intubation; for example, chest compressions, electrical therapy, intravenous access, or the administration of drugs. An important recent realization is that out-of-hospital endotracheal intubation may influence patient outcome by interacting with or affecting the execution of these simultaneous therapies. These observations have occurred in several disease groups. For example, after successful out-of-hospital endotracheal intubation, rescuers commonly perform ventilation manually (without the assistance of portable ventilators) using tactile feedback only. Consequently, out-of-hospital endotracheal intubation may result in unintended hyperventilation, which may be deleterious in certain conditions. In porcine models of hemorrhagic shock, Pepe et al 48, 49 found that increased respiratory rates (20 and 30 breaths/min) resulted in decreased systolic blood pressure and cardiac output, respectively. Davis et al50 showed that hyperventilation occurs frequently after out-of-hospital rapid-sequence intubation for traumatic brain injury, a condition in which hyperventilation can reduce cerebral perfusion. The investigators noted an association between hyperventilation and increased mortality.51 Aufderheide and Lurie52 and Aufderheide et al53 identified the same hyperventilation phenomenon in intubated cardiac arrest patients. Using physician responders to monitor out-of-hospital cardiac arrest victims, the authors also found that accidental hyperventilation raised intrathoracic pressure during chest compressions, thereby impeding coronary perfusion pressure, an important element for successful resuscitation.54 They observed that these episodes occurred despite the specific training of the paramedics in this study. Experts believe that control of intracranial pressure is important in the treatment of traumatic brain injury.50, 55, 56, 57 Physicians often use rapid sequence intubation to attenuate intracranial pressure response to the stress of endotracheal intubation.56 The aim to control intracranial pressure led to the proposal to replace nasotracheal intubation with rapid sequence intubation in these patients. However, the San Diego Rapid Sequence Intubation Trial found that adverse events such as inadvertent hyperventilation, oxygen desaturation, bradycardia, and increased mortality occurred with rapid sequence intubation approaches.20, 42, 51 Thus, efforts to precisely control one aspect of physiology during airway management disturbed other body systems. Although meriting additional study, these findings in different disease states suggest that unanticipated physiologic effects may offset the potential benefits of proper endotracheal intubation. These observations underscore our poor understanding of how current field airway management, oxygenation, ventilation, and other physiologic processes interact during the resuscitation of different disease states. How Do Paramedics Learn and Maintain Intubation Skills? Because the manner of intubation may affect patient outcome, a logical area of concern involves paramedic acquisition and maintenance of out-of-hospital endotracheal intubation skill. For example, in the Gausche et al22 study, paramedics did not perform pediatric out-of-hospital endotracheal intubation before the trial. In the San Diego Rapid Sequence Intubation Trial, paramedics received a 7-hour didactic session without supplemental live training.58 These factors may have reduced the potential benefit of out-of-hospital endotracheal intubation. Endotracheal intubation is a complex procedure, arguably more difficult when attempted in the uncontrolled out-of-hospital setting. Unlike physicians working in protected, stable, and well-illuminated settings (such as the operating room and ED), paramedics often attempt endotracheal intubation in awkward situations; for example, on the floor, in cramped rooms, or in the twisted metal of a motor vehicle.59, 60 Out-of-hospital patients are critically ill and often severely injured, and most would be considered “difficult” or high-risk intubations by in-hospital anesthesia standards.61 Given these challenges, one would expect paramedics to acquire and maintain endotracheal intubation skills well above minimum levels. However, paramedic endotracheal intubation training and clinical experience are relatively limited. For example, there is significant disparity between consensus procedural standards for paramedic students and other endotracheal intubation providers. The national paramedic curriculum requires students to perform 5 successful endotracheal intubations to graduate.62 In contrast, emergency medicine residents, anesthesiology residents, and nurse anesthetist trainees are expected to perform between 35 and 200 endotracheal intubations before graduating from their respective training programs.63, 64, 65, 66, 67, 68 Using data on 7,635 endotracheal intubations attempted by 802 paramedic trainees from 60 training programs, we found that across all clinical settings (operating room, field, ED, other in-hospital), paramedic students attempted a median of 7 endotracheal intubation (interquartile range 4-12).69 Using multivariable modeling, we predicted that paramedic students in this cohort required 15 to 20 endotracheal intubation encounters to attain baseline “proficiency” (predicted endotracheal intubation success threshold of 90%). Similar modeling efforts using cohorts of medical students, paramedic students, and anesthesia residents have identified even higher thresholds for attaining proficiency.63, 66, 70, 71 These observations suggest that the ideal level of baseline endotracheal intubation experience is much higher than the current national standard of 5 endotracheal intubations.62 Endotracheal intubation training in the controlled operating room setting is ideal, but according to these figures, the number of operating room cases needed to train paramedic students is formidable, approximately 80,000 operating room cases nationally each year (approximately 200 accredited paramedic training programs times approximately 20 graduating students/program/year times 20 endotracheal intubations/student/year=80,000 intubations/year).69 This estimate does not include students in nonaccredited paramedic programs or paramedics already in clinical practice. Furthermore, although increasing opportunities for operating room endotracheal intubation training is desired, paramedic training programs nationally have observed a general reduction in these opportunities, a phenomenon attributed to competition with other students, the widespread use of nonintubation techniques (such as the laryngeal mask airway), and anesthesiologists’ medicolegal concerns.72 Mannequins and human simulators provide opportunities for paramedic endotracheal intubation training, but only 1 study has evaluated how these platforms translate to clinical skill. In an effort occurring more than 20 years ago, Stewart et al2 assigned paramedics to different training strategies, including combinations of mannequin, animal, and operating room endotracheal intubation. Although the authors indicated no difference in clinical endotracheal intubation success rates between the groups on limited multivariable analysis, the unadjusted data suggested higher initial and ongoing endotracheal intubation success in the operating room–trained groups. A more recent effort by Hall et al73 compared paramedic operating room training with human simulator training. Although the authors stated equivalence between the 2 modalities, the evaluated outcome was operating room endotracheal intubation performance, not clinical out-of-hospital endotracheal intubation performance. Cadavers (ie, recently dead patients) have also been used for paramedic endotracheal intubation training, but only 1 study has evaluated the connection with clinical performance. Stratton et al74 compared mannequin with mannequin+cadaver training and found no difference in clinical endotracheal intubation performance between these groups. However, this inference was based on 60 paramedics, each performing a mean of only 3 endotracheal intubations. There are no direct comparisons of cadaver and operating room–based training. Cadavers were once widely used for teaching paramedic endotracheal intubation, but recent ethical concerns have curtailed learning opportunities on the recently dead.75, 76 Beyond baseline proficiency, regular clinical experience is likely an important element for maintaining endotracheal intubation skill. Studies of complex medical procedures (such as cardiac catheterization and bypass) suggest that centers and practitioners who perform these interventions frequently have improved survival and lower complication rates.77, 78, 79, 80 In Maine, Burton et al81 found that only 40% of paramedics attempted out-of-hospital endotracheal intubation annually, and only 1% to 2% of all paramedics performed 5 or more out-of-hospital endotracheal intubations annually. Separate from that study, we used 2003 statewide data from Pennsylvania, tallying the number of out-of-hospital endotracheal intubations performed by certified advanced life support rescuers (paramedics, out-of-hospital nurses, EMS physicians).82 We found that rescuers performed a median of only 1 out-of-hospital endotracheal intubation (interquartile range 0 to 3) during the study period; 39% performed no out-of-hospital endotracheal intubations, and 67% performed fewer than 2 out-of-hospital endotracheal intubations. Thus, contrary to common assumptions, most individual paramedics performed the procedure infrequently. Although the exact numbers needed to maintain out-of-hospital endotracheal intubation skill are unknown, these procedural frequency figures seem relatively low. The original models of out-of-hospital endotracheal intubations in Boston, Columbus, San Diego, and Pittsburgh were designed for small, closely supervised, highly skilled cadres of paramedics working in busy urban EMS systems and supported by intensive training.1, 2, 3, 4 Today, although many individual paramedics demonstrate exceptional endotracheal intubation skill, one must reconsider whether all paramedics nationally can attain the same level of excellence, given current limits in endotracheal intubation training and clinical experience. Can We Improve Out-of-Hospital Endotracheal Intubation? The current literature draws attention to many problematic aspects of out-of-hospital endotracheal intubation while offering few affirmations of current practice. Proposed solutions address only isolated aspects of the procedure, and none provide perfect or complete answers. For example, some have proposed that systematic use of waveform capnography could eliminate endotracheal tube misplacements in the out-of-hospital setting.39, 83, 84 Silvestri et al83 recently reviewed 213 out-of-hospital endotracheal intubations arriving at a Florida Level I trauma center. The authors found that the tube misplacement rate was zero percent when waveform capnography was used and 23.3% when waveform capnography was not used. However, only limited formal data describe the accuracy of these devices on cardiac arrests, which comprise most of the endotracheal intubations in the out-of-hospital setting.83, 85, 86, 87 Some directors have expanded the use of sedative or neuromuscular blocking agents to improve the out-of-hospital endotracheal intubation success of nonarrest patients.88 However, as discussed previously, the San Diego Rapid-Sequence Intubation Trial showed that important complications may result when these advanced techniques are introduced on a large-scale basis.20, 42 Because of its profound sedative effects and stable hemodynamic profile, some EMS systems have used etomidate alone (without neuromuscular blockade) to facilitate out-of-hospital endotracheal intubation of nonarrest patients.89, 90 However, a recent randomized controlled trial comparing etomidate-only with midazolam-only facilitated cases found no difference in out-of-hospital endotracheal intubation success rates.91 The observed etomidate endotracheal intubation success rate in this trial was 76% (95% CI 65% to 87%), which may be below desired success thresholds. Furthermore, the authors performed only a limited outcomes analysis. Some EMS services have improved procedural experience and proficiency by using fewer targeted-response paramedics.5, 92, 93 In the original implementation effort by DeLeo, the investigator purposely constrained the number of paramedic units to maximize out-of-hospital endotracheal intubation procedural exposure.3 Although feasible in dense urban settings, these strategies may not be possible in remote rural areas where ambulances already cover large geographic distances. These approaches are also at odds with the efforts of communities seeking to increase the number of paramedics in their regions.94 In the spirit of seeking system-level improvements, one cannot ignore the question, Should we intubate at all? For apneic or near-apneic patients, alternate airways such as the Combitube (esophageal-tracheal twin-lumen airway device; Kendall, Inc., Mansfield, MA) and laryngeal mask airway (LMA North America, San Diego, CA) have appealing characteristics and are supported by some data.95, 96, 97, 98 These devices are conceptually simpler than endotracheal intubation, easier to insert than endotracheal tubes, require less training, and are less subject to skill decay.71, 96, 99, 100, 101 These devices have been extensively used as primary and secondary airway management devices.102, 103, 104 There is wide experience with the use of these devices by nonphysicians and even basic-level rescuers.97, 101, 105, 106, 107, 108, 109, 110, 111, 112 Combitubes and laryngeal mask airways offer ventilation and oxygenation comparable with endotracheal intubation in controlled and field settings.96, 100, 113, 114, 115 Current advanced cardiac life support guidelines recommend the use of these devices when rescuers have only limited endotracheal intubation experience.116 Combitubes and laryngeal mask airways have important adverse effects, limitations, and concerns, including many similar to those of endotracheal intubation.45, 117, 118, 119, 120, 121, 122 Most important, their links to patient outcome have not been defined. Before Combitubes and laryngeal mask airways can formally replace endotracheal intubation, we must perform careful systematic evaluations to verify their safety and effectiveness. Conclusion The current literature highlights shortcomings associated with out-of-hospital endotracheal intubation. Few studies affirm current practice. Few studies have demonstrated improved outcome from out-of-hospital endotracheal intubation in any disease group, and several studies describe worsened outcomes. In many studies, adverse events and errors associated with out-of-hospital endotracheal intubation are frequent. Out-of-hospital endotracheal intubation may inadvertently interact with other physiologic processes key to optimizing resuscitation. Significant system-level barriers limit opportunities for endotracheal intubation training and clinical experience. Scientists, medical directors, and clinicians must strive to better understand and ultimately improve this key intervention. References 1. 1Jacobs LM, Berrizbeitia LD, Bennett B, et al.. Endotracheal intubation in the prehospital phase of emergency medical care. JAMA. 1983;250:2175–2177. MEDLINE 2. 2Stewart RD, Paris PM, Winter PM, et al.. Field endotracheal intubation by paramedical personnel (success rates and complications). Chest. 1984;85:341–345. MEDLINE 3. 3DeLeo BC. Endotracheal intubation by rescue squad personnel. Heart Lung. 1977;6:851–854. MEDLINE 4. 4Guss DA, Posluszny M. Paramedic orotracheal intubation (a feasibility study). Am J Emerg Med. 1984;2:399–401. MEDLINE | CrossRef 5. 5Pepe PE, Warnke WJ. Emergency medical services and systems of out-of-hospital resuscitation. In: Paradis NA, Halperin HR, Nowack RM editor. Cardiac Arrest (The Science and Practice of Resuscitation Medicine). Baltimore, MD: Williams and Wilkins; 1996;p. 581–596. 6. 6Cummins RO, Austin D, Graves JR, et al.. Ventilation skills of emergency medical technicians (a teaching challenge for emergency medicine). Ann Emerg Med. 1986;15:1187–1192. Abstract | Abstract + References | PDF (630 KB) | MEDLINE | CrossRef 7. 7Smith JP, Bodai BI, Seifkin A, et al.. The esophageal obturator airway (a review). JAMA. 1983;250:1081–1084. MEDLINE 8. 8Bryson TK, Benumof JL, Ward CF. The esophageal obturator airway (a clinical comparison to ventilation with a mask and oropharyngeal airway). Chest. 1978;74:537–539. MEDLINE 9. 9Michael TA. Comparison of the esophageal obturator airway and endotracheal intubation in prehospital ventilation during CPR. Chest. 1985;87:814–819. MEDLINE 10. 10Hess D, Baran C. Ventilatory volumes using mouth-to-mouth, mouth-to-mask, and bag-valve-mask techniques. Am J Emerg Med. 1985;3:292–296. MEDLINE | CrossRef 11. 11Elling R, Politis J. An evaluation of emergency medical technicians’ ability to use manual ventilation devices. Ann Emerg Med. 1983;12:765–768. Abstract | Abstract + References | PDF (409 KB) | MEDLINE | CrossRef 12. 12Hankins DG, Carruthers N, Frascone RJ, et al.. Complication rates for the esophageal obturator airway and endotracheal tube in the prehospital setting. Prehospital Disaster Med. 1993;8:117–121. 13. 13Smith JP, Bodai BI, Aubourg R, et al.. A field evaluation of the esophageal obturator airway. J Trauma. 1983;23:317–321. MEDLINE 14. 14Yancey W, Wears R, Kamajian G, et al.. Unrecognized tracheal intubation (a complication of the esophageal obturator airway). Ann Emerg Med. 1980;9:18–20. Abstract | Abstract + References | PDF (1101 KB) | MEDLINE | CrossRef 15. 15Gertler JP, Cameron DE, Shea K, et al.. The esophageal obturator airway (obturator or obtundator?). J Trauma. 1985;25:424–426. MEDLINE 16. 16Pepe PE, Copass MK, Joyce TH. Prehospital endotracheal intubation (rationale for training emergency medical personnel). Ann Emerg Med. 1985;14:1085–1092. Abstract | Abstract + References | PDF (1004 KB) | MEDLINE | CrossRef 17. 17American Heart Association. Guidelines for cardiopulmonary resuscitation and emergency cardiac care: Emergency Cardiac Care Committee and subcommittees, American Heart Association, part III: adult advanced cardiac life support. JAMA. 1992;268:2199–2241. MEDLINE 18. 18Spaite DW, Maio R, Garrison HG, et al.. Emergency Medical Services Outcomes Project (EMSOP) II (developing the foundation and conceptual models for out-of-hospital outcomes research). Ann Emerg Med. 2001;37:657–663. Abstract | Full Text | PDF (86 KB) | MEDLINE | CrossRef 19. 19Bulger EM, Copass MK, Sabath DR, et al.. The use of neuromuscular blocking agents to facilitate prehospital intubation does not impair outcome after traumatic brain injury. J Trauma. 2005;58:718–723. MEDLINE 20. 20Davis DP, Hoyt DB, Ochs M, et al.. The effect of paramedic rapid sequence intubation on outcome in patients with severe traumatic brain injury. J Trauma. 2003;54:444–453. MEDLINE 21. 21Davis DP, Peay J, Sise MJ, et al.. The impact of prehospital endotracheal intubation on outcome in moderate to severe traumatic brain injury. J Trauma. 2005;58:933–939. MEDLINE 22. 22Gausche M, Lewis RJ, Stratton SJ, et al.. Effect of out-of-hospital pediatric endotracheal intubation on survival and neurological outcome (a controlled clinical trial). JAMA. 2000;283:783–790. MEDLINE | CrossRef 23. 23Lockey D, Davies G, Coats T. Survival of trauma patients who have prehospital tracheal intubation without anaesthesia or muscle relaxants (observational study). [abstract]BMJ. 2001;323:141. MEDLINE | CrossRef 24. 24Murray JA, Demetriades D, Berne TV, et al.. Prehospital intubation in patients with severe head injury. J Trauma. 2000;49:1065–1070. MEDLINE 25. 25Sloane C, Vilke GM, Chan TC, et al.. Rapid sequence intubation in the field versus hospital in trauma patients. J Emerg Med. 2000;19:259–264. Abstract | Full Text | PDF (108 KB) | MEDLINE | CrossRef 26. 26Stockinger ZT, McSwain NE. Prehospital endotracheal intubation for trauma does not improve survival over bag-valve-mask ventilation. J Trauma. 2004;56:531–536. MEDLINE 27. 27Wang HE, Peitzman AB, Cassidy LD, et al.. Out-of-hospital endotracheal intubation and outcome after traumatic brain injury. Ann Emerg Med. 2004;44:439–450. Abstract | Full Text | PDF (170 KB) 28. 28Winchell RJ, Hoyt DB. Endotracheal intubation in the field improves survival in patients with severe head injury (Trauma Research and Education Foundation of San Diego). Arch Surg. 1997;132:592–597. MEDLINE 29. 29Bochicchio GV, Ilahi O, Joshi M, et al.. Endotracheal intubation in the field does not improve outcome in trauma patients who present without an acutely lethal traumatic brain injury. J Trauma. 2003;54:307–311. MEDLINE 30. 30Christensen EF, Hoyer CC. Prehospital tracheal intubation in severely injured patients (a Danish observational study). BMJ. 2003;327:533–534. CrossRef 31. 31Cooper A, DiScala C, Foltin G, et al.. Prehospital endotracheal intubation for severe head injury in children (a reappraisal). Semin Pediatr Surg. 2001;10:3–6. Abstract | Abstract + References | MEDLINE 32. 32DiRusso SM, Sullivan T, Risucci D, et al.. Intubation of pediatric trauma patients in the field (predictor of negative outcome despite risk stratification). J Trauma. 2005;59:84–90. MEDLINE 33. 33Suominen P, Baillie C, Kivioja A, et al.. Intubation and survival in severe paediatric blunt head injury. Eur J Emerg Med. 2000;7:3–7. MEDLINE 34. 34Hudak AM, Caesar RR, Frol AB, et al.. Functional outcome scales in traumatic brain injury (a comparison of the Glasgow Outcome Scale (extended) and the Functional Status Examination). J Neurotrauma. 2005;22:1319–1326. MEDLINE | CrossRef 35. 35Stineman MG, Shea JA, Jette A, et al.. The Functional Independence Measure (tests of scaling assumptions, structure, and reliability across 20 diverse impairment categories). Arch Phys Med Rehabil. 1996;77:1101–1108. Abstract | Abstract + References | PDF (948 KB) | MEDLINE | CrossRef 36. 36Garrison HG, Maio RF, Spaite DW, et al.. Emergency Medical Services Outcomes Project III (EMSOP III) (the role of risk adjustment in out-of-hospital outcomes research). Ann Emerg Med. 2002;40:79–88. Abstract | Full Text | PDF (73 KB) | MEDLINE | CrossRef 37. 37Kohn LT, Corrigan J, Donaldson MS. To Err is Human (Building a Safer Health System). Washington, DC: National Academy Press; 2000;. 38. 38O’Connor RE, Slovis CM, Hunt RC, et al.. Eliminating errors in emergency medical services (realities and recommendations). Prehosp Emerg Care. 2002;6:107–113. MEDLINE 39. 39Katz SH, Falk JL. Misplaced endotracheal tubes by paramedics in an urban emergency medical services system. Ann Emerg Med. 2001;37:32–37. Abstract | Full Text | PDF (68 KB) | MEDLINE | CrossRef 40. 40Jemmett ME, Kendal KM, Fourre MW, et al.. Unrecognized misplacement of endotracheal tubes in a mixed urban to rural emergency medical services setting. Acad Emerg Med. 2003;10:961–965. MEDLINE | CrossRef 41. 41Jones JH, Murphy MP, Dickson RL, et al.. Emergency physician-verified out-of-hospital intubation (miss rates by paramedics). Acad Emerg Med. 2004;11:707–709. MEDLINE | CrossRef 42. 42Dunford JV, Davis DP, Ochs M, et al.. Incidence of transient hypoxia and pulse rate reactivity during paramedic rapid sequence intubation. Ann Emerg Med. 2003;42:721–728. Abstract | Full Text | PDF (187 KB) | MEDLINE | CrossRef 43. 43Ehrlich PF, Seidman PS, Atallah O, et al.. Endotracheal intubations in rural pediatric trauma patients. J Pediatr Surg. 2004;39:1376–1380. Abstract | Full Text | PDF (65 KB) | CrossRef 44. 44Wang HE, Lave JR, Sirio CA, et al.. Paramedic intubation errors (isolated events or symptoms of larger problems?). Health Aff (Millwood). 2006;25:501–509. CrossRef 45. 45Jaeger K, Ruschulte H, Osthaus A, et al.. Tracheal injury as a sequence of multiple attempts of endotracheal intubation in the course of a preclinical cardiopulmonary resuscitation. Resuscitation. 2000;43:147–150. MEDLINE | CrossRef 46. 46Mizelle HL, Rothrock SG, Silvestri S, et al.. Preventable morbidity and mortality from prehospital paralytic assisted intubation (can we expect outcomes comparable to hospital-based practice?). Prehosp Emerg Care. 2002;6:472–475. MEDLINE 47. 47Bledsoe BE, Porter RS, Cherry RA. Paramedic Care (Principles and Practice). Upper Saddle River, NJ: Prentice-Hall; 2000;. 48. 48Pepe PE, Raedler C, Lurie KG, et al.. Emergency ventilatory management in hemorrhagic states (elemental or detrimental?). J Trauma. 2003;54:1048–1055. MEDLINE 49. 49Pepe PE, Roppolo LP, Fowler RL. The detrimental effects of ventilation during low-blood-flow states. Curr Opin Crit Care. 2005;11:212–218. MEDLINE | CrossRef 50. 50Brain Trauma Foundation. Management and prognosis of severe traumatic brain injury. Available at: http://www2.braintrauma.org/guidelines/dow..._management.pdf. Accessed June 30, 2005. 51. 51Davis DP, Dunford JV, Poste JC, et al.. The impact of hypoxia and hyperventilation on outcome after paramedic rapid sequence intubation of severely head-injured patients. J Trauma. 2004;57:1–8. MEDLINE 52. 52Aufderheide TP, Lurie KG. Death by hyperventilation (a common and life-threatening problem during cardiopulmonary resuscitation). Crit Care Med. 2004;32:S345–S351. 53. 53Aufderheide TP, Sigurdsson G, Pirrallo RG, et al.. Hyperventilation-induced hypotension during cardiopulmonary resuscitation. Circulation. 2004;109:1960–1965. CrossRef 54. 54Paradis NA, Martin GB, Rivers EP, et al.. Coronary perfusion pressure and the return of spontaneous circulation in human cardiopulmonary resuscitation. JAMA. 1990;263:1106–1113. MEDLINE 55. 55Biros MH, Heegaard W. Prehospital and resuscitative care of the head-injured patient. Curr Opin Crit Care. 2001;7:444–449. MEDLINE 56. 56Walls RM. Rapid-sequence intubation in head trauma. Ann Emerg Med. 1993;22:1008–1013. Abstract | Abstract + References | PDF (628 KB) | MEDLINE | CrossRef 57. 57Brain Trauma Foundation. Guidelines for prehospital management of traumatic brain injury. Available at: http://www2.braintrauma.org/guidelines/dow...prehospital.pdf. Accessed June 30, 2005. 58. 58Ochs M, Davis D, Hoyt D, et al.. Paramedic-performed rapid sequence intubation of patients with severe head injuries. Ann Emerg Med. 2002;40:159–167. Abstract | Full Text | PDF (70 KB) | MEDLINE | CrossRef 59. 59Tesler J, Rucker J, Sommer D, et al.. Rescuer position for tracheal intubation on the ground. Resuscitation. 2003;56:83–89. MEDLINE | CrossRef 60. 60Adnet F, Cydulka RK, Lapandry C. Emergency tracheal intubation of patients lying supine on the ground (influence of operator body position). Can J Anaesth. 1998;45:266–269. MEDLINE 61. 61Benumof JL. Management of the difficult adult airway (with special emphasis on awake tracheal intubation). Anesthesiology. 1991;75:1087–1110. MEDLINE 62. 62National Highway Traffic Safety Administration. Emergency medical technician paramedic: national standard curriculum (EMT-P). Available at: http://www.nhtsa.dot.gov/people/injury/ems/EMT-P. Accessed October 8, 2004. 63. 63de Oliveira Filho GR. The construction of learning curves for basic skills in anesthetic procedures (an application for the cumulative sum method). Anesth Analg. 2002;95:411–416. MEDLINE 64. 64Accreditation Council for Graduate Medical Education: Emergency medicine: guidelines for procedures and resuscitations. Available at: http://www.acgme.org/acWebsite/RRC_110/110...delines.asp#res. Accessed November 12, 2004. 65. 65Council on Accreditation of Nurse Anesthesia Educational Programs (Standards for Accreditation of Nurse Anesthesia Educational Programs). 2004;Park Ridge, IL. 66. 66Konrad C, Schupfer G, Wietlisbach M, et al.. Learning manual skills in anesthesiology (is there a recommended number of cases for anesthetic procedures?). Anesth Analg. 1998;86:635–639. MEDLINE 67. 67Kopacz DJ, Neal JM, Pollock JE. The regional anesthesia “learning curve” (what is the minimum number of epidural and spinal blocks to reach consistency?). Reg Anesth. 1996;21:182–190. MEDLINE 68. 68Charuluxananan S, Kyokong O, Somboonviboon W, et al.. Learning manual skills in spinal anesthesia and orotracheal intubation (is there any recommended number of cases for anesthesia residency training program?). J Med Assoc Thai. 2001;84:S251–S255. 69. 69Wang HE, Seitz SR, Hostler D, et al.. Defining the “learning curve” for paramedic student endotracheal intubation. Prehosp Emerg Care. 2005;9:156–162. MEDLINE | CrossRef 70. 70Mulcaster JT, Mills J, Hung OR, et al.. Laryngoscopic intubation (learning and performance). Anesthesiology. 2003;98:23–27. MEDLINE | CrossRef 71. 71Tiah L, Wong E, Chen MF, et al.. Should there be a change in the teaching of airway management in the medical school curriculum?. Resuscitation. 2005;64:87–91. Abstract | Full Text | PDF (159 KB) | MEDLINE | CrossRef 72. 72Johnston BD, Seitz SR, Wang HE. National limitations in paramedic student operating room training for paramedic endotracheal intubation. [abstract]Prehosp Emerg Care. 2006;10:107. MEDLINE | CrossRef 73. 73Hall RE, Plant JR, Bands CJ, et al.. Human patient simulation is effective for teaching paramedic students endotracheal intubation. Acad Emerg Med. 2005;12:850–855. CrossRef 74. 74Stratton SJ, Kane G, Gunter CS, et al.. Prospective study of manikin-only versus manikin and human subject endotracheal intubation training of paramedics. Ann Emerg Med. 1991;20:1314–1318. Abstract | Abstract + References | PDF (1270 KB) | MEDLINE | CrossRef 75. 75Orlowski JP, Kanoti GA, Mehlman MJ. The ethics of using newly dead patients for teaching and practicing intubation techniques. N Engl J Med. 1988;319:439–441. MEDLINE 76. 76Morag RM, DeSouza S, Steen PA, et al.. Performing procedures on the newly deceased for teaching purposes (what if we were to ask?). Arch Intern Med. 2005;165:92–96. MEDLINE | CrossRef 77. 77Luft HS. Hospital Volume, Physician Volume, and Patient Outcomes (Assessing the Evidence). Ann Arbor, MI: Health Administration Press; 1990;. 78. 78Konvolinka CW, Copes WS, Sacco WJ. Institution and per-surgeon volume versus survival outcome in Pennsylvania’s trauma centers. Am J Surg. 1995;170:333–340. Abstract | Abstract + References | PDF (992 KB) | MEDLINE | CrossRef 79. 79Kastrati A, Neumann FJ, Schomig A. Operator volume and outcome of patients undergoing coronary stent placement. J Am Coll Cardiol. 1998;32:970–976. Abstract | Full Text | PDF (117 KB) | MEDLINE | CrossRef 80. 80IJsselmuiden S, Kiemeneij F, Tangelder G, et al.. Impact of operator volume on overall major adverse cardiac events following direct coronary stent implantation versus stenting after predilatation. Int J Cardiovasc Intervent. 2004;6:5–12. MEDLINE | CrossRef 81. 81Burton JH, Baumann MR, Maoz T, et al.. Endotracheal intubation in a rural EMS state (procedure utilization and impact of skills maintenance guidelines). Prehosp Emerg Care. 2003;7:352–356. MEDLINE 82. 82Wang HE, Kupas DF, Hostler D, et al.. Procedural experience with out-of-hospital endotracheal intubation. Crit Care Med. 2005;33:1718–1721. MEDLINE | CrossRef 83. 83Silvestri S, Ralls GA, Krauss B, et al.. The effectiveness of out-of-hospital use of continuous end-tidal carbon dioxide monitoring on the rate of unrecognized misplaced intubation within a regional emergency medical services system. Ann Emerg Med. 2005;45:497–503. Abstract | Full Text | PDF (123 KB) | CrossRef 84. 84O’Connor RE, Swor RA. Verification of endotracheal tube placement following intubation (National Association of EMS Physicians Standards and Clinical Practice Committee). Prehosp Emerg Care. 1999;3:248–250. MEDLINE 85. 85American Society for Anesthesiology. Standards for basic anesthetic monitoring. Available at: http://www.asahq.org/publicationsAndServic...tandards/02.pdf. Accessed July 2, 2005. 86. 86Grmec S. Comparison of three different methods to confirm tracheal tube placement in emergency intubation. Intensive Care Med. 2002;28:701–704. MEDLINE | CrossRef 87. 87Tanigawa K, Takeda T, Goto E, et al.. The efficacy of esophageal detector devices in verifying tracheal tube placement (a randomized cross-over study of out-of-hospital cardiac arrest patients). Anesth Analg. 2001;92:375–378. MEDLINE 88. 88Wayne MA, Friedland E. Prehospital use of succinylcholine (a 20-year review). Prehosp Emerg Care. 1999;3:107–109. MEDLINE 89. 89Bozeman WP, Young S. Etomidate as a sole agent for endotracheal intubation in the prehospital air medical setting. Air Med J. 2002;21:32–35. MEDLINE | CrossRef 90. 90Reed DB, Snyder G, Hogue TD. Regional EMS experience with etomidate for facilitated intubation. Prehosp Emerg Care. 2002;6:50–53. MEDLINE 91. 91Jacoby J, Cesta M, McGee J, et al.. Etomidate versus midazolam for pre-hospital intubation (a prospective randomized trial). Ann Emerg Med. 2003;42:S48. 92. 92Persse DE, Key CB, Bradley RN, et al.. Cardiac arrest survival as a function of ambulance deployment strategy in a large urban emergency medical services system. Resuscitation. 2003;59:97–104. MEDLINE | CrossRef 93. 93Stout J, Pepe PE, Mosesso VN. All-advanced life support vs tiered-response ambulance systems. Prehosp Emerg Care. 2000;4:1–6. MEDLINE 94. 94Davis R. Paramedics not always the saviors of cardiac-arrest patients. USA Today. 2005;March 2. Available at: http://www.usatoday.com/news/health/2005-0...ems-cover_x.htm. Accessed August 8, 2005.. 95. 95Deakin CD, Peters R, Tomlinson P, et al.. Securing the prehospital airway (a comparison of laryngeal mask insertion and endotracheal intubation by UK paramedics). Emerg Med J. 2005;22:64–67. CrossRef 96. 96Rumball CJ, MacDonald D. The PTL, Combitube, laryngeal mask, and oral airway (a randomized prehospital comparative study of ventilatory device effectiveness and cost-effectiveness in 470 cases of cardiorespiratory arrest). Prehosp Emerg Care. 1997;1:1–10. MEDLINE 97. 97Atherton GL, Johnson JC. Ability of paramedics to use the Combitube in prehospital cardiac arrest. Ann Emerg Med. 1993;22:1263–1268. Abstract | Abstract + References | PDF (547 KB) | MEDLINE | CrossRef 98. 98Agro F, Frass M, Benumof JL, et al.. Current status of the Combitube (a review of the literature). J Clin Anesth. 2002;14:307–314. Abstract | Full Text | PDF (263 KB) | MEDLINE | CrossRef 99. 99Rabitsch W, Schellongowski P, Staudinger T, et al.. Comparison of a conventional tracheal airway with the Combitube in an urban emergency medical services system run by physicians. Resuscitation. 2003;57:27–32. MEDLINE | CrossRef 100. 100Staudinger T, Brugger S, Watschinger B, et al.. Emergency intubation with the Combitube (comparison with the endotracheal airway). Ann Emerg Med. 1993;22:1573–1575. Abstract | Abstract + References | PDF (271 KB) | MEDLINE | CrossRef 101. 101Reinhart DJ, Simmons G. Comparison of placement of the laryngeal mask airway with endotracheal tube by paramedics and respiratory therapists. Ann Emerg Med. 1994;24:260–263. Abstract | Full Text | MEDLINE 102. 102Davis DP, Valentine C, Ochs M, et al.. The Combitube as a salvage airway device for paramedic rapid sequence intubation. Ann Emerg Med. 2003;42:697–704. Abstract | Full Text | PDF (101 KB) | MEDLINE | CrossRef 103. 103O’Malley RN, O’Malley GF, Ochi G. Emergency medicine in Japan. Ann Emerg Med. 2001;38:441–446. Abstract | Full Text | PDF (67 KB) | MEDLINE | CrossRef 104. 104Tanigawa K, Shigematsu A. Choice of airway devices for 12,020 cases of nontraumatic cardiac arrest in Japan. Prehosp Emerg Care. 1998;2:96–100. MEDLINE 105. 105Pennant JH, Walker MB. Comparison of the endotracheal tube and laryngeal mask in airway management by paramedical personnel. Anesth Analg. 1992;74:531–534. MEDLINE 106. 106Dorges V, Wenzel V, Knacke P, et al.. Comparison of different airway management strategies to ventilate apneic, nonpreoxygenated patients. Crit Care Med. 2003;31:800–804. MEDLINE 107. 107Martin PD, Cyna AM, Hunter WA, et al.. Training nursing staff in airway management for resuscitation (a clinical comparison of the facemask and laryngeal mask). Anaesthesia. 1993;48:33–37. MEDLINE 108. 108Alexander R, Hodgson P, Lomax D. A comparison of the laryngeal mask airway and Guedel airway, bag and facemask for manual ventilation following formal training. Anaesthesia. 1993;48:231–234. MEDLINE 109. 109Davies PR, Tighe SQ, Greenslade GL, et al.. Laryngeal mask airway and tracheal tube insertion by unskilled personnel. Lancet. 1990;336:977–979. MEDLINE | CrossRef 110. 110Ochs M, Vilke GM, Chan TC, et al.. Successful prehospital airway management by EMT-Ds using the Combitube. Prehosp Emerg Care. 2000;4:333–337. MEDLINE | CrossRef 111. 111Rumball C, Macdonald D, Barber P, et al.. Endotracheal intubation and esophageal tracheal Combitube insertion by regular ambulance attendants (a comparative trial). Prehosp Emerg Care. 2004;8:15–22. MEDLINE 112. 112Lefrancois DP, Dufour DG. Use of the esophageal tracheal Combitube by basic emergency medical technicians. Resuscitation. 2002;52:77–83. MEDLINE 113. 113Frass M, Frenzer R, Rauscha F, et al.. Ventilation with the esophageal tracheal Combitube in cardiopulmonary resuscitation (promptness and effectiveness). Chest. 1988;93:781–784. MEDLINE 114. 114Kurola J, Harve H, Kettunen T, et al.. Airway management in cardiac arrest (comparison of the laryngeal tube, tracheal intubation and bag-valve mask ventilation in emergency medical training). Resuscitation. 2004;61:149–153. Abstract | Full Text | PDF (60 KB) | MEDLINE 115. 115Frass M, Rodler S, Frenzer R, et al.. Esophageal tracheal Combitube, endotracheal airway, and mask (comparison of ventilatory pressure curves). J Trauma. 1989;29:1476–1479. MEDLINE 116. 116Barnes TA, Macdonald D, Nolan J, et al.. Cardiopulmonary resuscitation and emergency cardiovascular care (airway devices). Ann Emerg Med. 2001;37:S145–S151. Abstract | Full Text | PDF (83 KB) 117. 117Stoppacher R, Teggatz JR, Jentzen JM. Esophageal and pharyngeal injuries associated with the use of the esophageal-tracheal Combitube. J Forensic Sci. 2004;49:586–591. MEDLINE 118. 118Vezina D, Lessard MR, Bussieres J, et al.. Complications associated with the use of the esophageal-tracheal Combitube. Can J Anaesth. 1998;45:76–80. MEDLINE 119. 119Haslam N, Campbell GC, Duggan JE. Gastric rupture associated with use of the laryngeal mask airway during cardiopulmonary resuscitation. BMJ. 2004;329:1225–1226. 120. 120Ufberg JW, Bushra JS, Karras DJ, et al.. Aspiration of gastric contents (association with prehospital intubation). Am J Emerg Med. 2005;23:379–382. Abstract | Full Text | PDF (81 KB) | MEDLINE 121. 121Stone BJ, Chantler PJ, Baskett PJ. The incidence of regurgitation during cardiopulmonary resuscitation (a comparison between the bag valve mask and laryngeal mask airway). Resuscitation. 1998;38:3–6. MEDLINE 122. 122Hagberg CA, Vartazarian TN, Chelly JE, et al.. The incidence of gastroesophageal reflux and tracheal aspiration detected with pH electrodes is similar with the laryngeal mask airway and esophageal tracheal Combitube (a pilot study). Can J Anaesth. 2004;51:243–249. MEDLINE Department of Emergency Medicine, University of Pittsburgh School of Medicine, Pittsburgh, PA. Address for correspondence: Henry E. Wang, MD, MPH, University of Pittsburgh School of Medicine, Department of Emergency Medicine, 230 McKee Place, Suite 400, Pittsburgh, PA, 15213; 412-647-4925, fax 412-647-6999 Supervising editor: Robert K. Knopp, MD Funding and support: Dr. Wang is supported by Clinical Scientist Development Award K08-HS013628 from the Agency for Healthcare Research and Quality, Rockville, MD. Reprints not available from the authors. PII: S0196-0644(06)00075-8 doi:10.1016/j.annemergmed.2006.01.016 © 2006 American College of Emergency Physicians. Published by Elsevier Inc. All rights reserved.
-
-
Hello Everyone, Here's an interesting editorial, what do you all think? (For Rich and Poor @ the Message Is Still “Dial 9-1-1”: But Is It Getting Through? For Rich and Poor, the Message Is Still “Dial 9-1-1”: But Is It Getting Through? [Editorial) Scott, Phillip A. MD, FAHA From the University of Michigan, Department of Emergency Medicine, Ann Arbor, MI. The opinions in this editorial are not necessarily those of the editors or of the American Heart Association. Correspondence and reprint requests to Phillip A. Scott, University of Michigan, Department of Emergency Medicine, 1500 E Medical Center Dr, TC B1354, Box 0303, Ann Arbor, MI 48109. E-mail phillip.scott@umich.edu See related article, pages 1508–1513.] The mantra for public service announcements on stroke has included 911/Emergency Medical System (EMS) use as the first point of contact for patients with stroke symptoms for over 10 years. The rationale behind this is clear. As with chest pain and acute myocardial infarction, 911/EMS use by patients with symptoms of stroke represents an early component in the Chain of Recovery.1 Furthermore, a single message links the urgency of “heart attack” and “brain attack”: reinforcing and directing action by the lay public. Though the benefits of 911/EMS use accrue in both the prehospital and in-hospital settings, it is unclear whether this message is getting through. In the prehospital arena, stroke patients using 911/EMS arrive to the emergency department faster in comparison to those calling their primary physician or driving to a hospital or primary care site directly. There is also a strong association between EMS use and shorter time periods from symptom onset to hospital arrival—though this likely reflects a greater sense of urgency on the patient’s or bystander’s part rather than reduced transport times. Additionally, almost 20% of patients with stroke symptoms have acute medical conditions requiring paramedic-level interventions in the field, including emergent airway management.2 In the hospital setting, a strong association exists between EMS arrival and reduced time to initial physician evaluation, CT imaging and neurologic consultation and evaluation. In this issue of Stroke, Kleindorfer et al extend our knowledge of EMS use by patients with stroke in their report on the association of socioeconomic status (SES) and demographics on differences in prehospital transport time for patients with stroke.3 Using data from the Greater Cincinnati/Northern Kentucky Stroke study, the authors performed retrospective analyses at the census tract level using Geographic Information Systems (GIS) mapping with current census and geocoding data for patients with the onset of stroke or TIA while at home. They have made appropriate adjustments to account for the potential effects of increased hospital proximity which could reasonably be found in low SES groups in a dense urban core and present well-defined rules for the management of data conflicts. In their study, only 38% of eligible patients were identified as arriving via EMS, a figure at the lower end of the range reported from other post-thrombolytic era studies (29% to 65%). In a geographic area with a long history of an active stroke program and substantial, long-term, professional and public education, this raises concerns that the public message to call 911 in response to stroke symptoms may be missing the mark. An alternate explanation may be the study’s exclusion of patients with stroke onset at locations other than their home (where a greater proportion of subjects may be alone). This resulted in a selection bias, a limitation the authors acknowledge. Even after making reasonable allowances for these limitations, though, concern remains regarding the effectiveness of national and local public stroke education efforts. Data from the DASH II study indicated that knowledge of stroke symptoms alone was insufficient to increase EMS use in stroke patients (odds ratio 0.63, 95% CI, 0.40 to 0.98) and that interventions to achieve this goal should stress the urgency of stroke.4 EMS activation through 911 appears to be a function primarily of individuals other than the patient with data suggesting 62% to 95% of 911 calls are made by a family member, caregiver, coworker or other bystander. Thus, public service messages should broadly target not only those at risk but also families, friends and coworkers. Recently presented data evaluating local TV reporting of medical news across the top 50 US media markets found only 0.7% of 1799 stories were unique stroke stories. Of the 13 stroke stories, 75% implied that receiving treatment within 7 to 14 hours was effective.5 The optimum method, message and medium to maximize the impact of public education to increase 911 use in patients with stroke symptoms remain to be identified and are worthy of further study. Encouragingly, the study authors found SES had no significant impact on total EMS time (initial 911 contact to hospital arrival) and that average EMS transport times were very brief (mean dispatch-to-arrival time of 34 minutes). Small (5 minute), statistically significant delays in total EMS time were identified for blacks, consistent with previous findings of discrepancies in access to healthcare in minority populations. Importantly, the article also provides new information as to the average direct-line distance stroke patients travel via ambulance to reach hospital-based care in this time interval. With the emergence of designated stroke centers, and subsequent potential regionalization of stroke care, this provides key data for the efficient design of EMS protocols allowing delivery of patients to centers with acute treatment capability. The promise of prehospital systems remains unfulfilled, and each link in the Chain of Recovery must be optimized to enhance the effectiveness of acute stroke therapy. Educational methods to increase patient action in accessing 911 after onset of symptoms should be sought. Though only minimal improvements in overall EMS time appear feasible, it may be possible to more effectively use transport time. This potentially includes the greater use of stroke screening tools such as the Los Angeles Prehospital Stroke Screen, Cincinnati Prehospital Stroke Scale or FAST (Face, Arm, Speech, Time) to provide earlier advance notice to receiving hospitals and stroke teams. The feasibility of neuroprotective agent delivery in the field has been demonstrated and may offer substantial time savings over the in-hospital setting.6,7 Finally, the design of regional EMS systems to leverage the impact of stroke centers should be sought. The findings of Kleindorfer et al indicate much work remains in getting the message, and the systems, right. Acknowledgments This work was supported in part by the following research grants: National Institutes of Health/National Institutes of Neurological Disorders and Stroke 1 RO1 NS050372, MDCH (Michigan Department of Community Health) N007058. References 1. Pepe PE, Zachariah BS, Sayre MR, Floccare D. Ensuring the chain of recovery for stroke in your community. Acad Emerg Med. 1998;5:352–358. SFX Bibliographic Links Library Holdings [Context Link] 2. Kothari R, Barsan W, Brott T, Broderick J, Ashbrock S. Frequency and accuracy of prehospital diagnosis of acute stroke. Stroke. 1995;26:937–941. Ovid Full Text Bibliographic Links Library Holdings [Context Link] 3. Kleindorfer DO, Lindsell CJ, Broderick JP, Flaherty ML, Woo D, Ewing I, Schmit P, Moomaw C, Alwell K, Pancioli A, Jauch E, Khoury J, Miller R, Schneider A, Kissela BM. Community socioeconomic status and Prehospital times in acute stroke and TIA: Do poorer patients have longer delays from 911 call to the emergency department. Stroke. 2006;37:1508–1513. Ovid Full Text Bibliographic Links Library Holdings [Context Link] 4. Schroeder EB, Rosamond WD, Morris DL, Evenson KR, Hinn AR. Determinants of use of emergency medical services in a population with stroke symptoms: the Second Delay in Accessing Stroke Healthcare (DASH II) Study. Stroke. 2000;31:2591–2596. Ovid Full Text Bibliographic Links Library Holdings [Context Link] 5. Pribble J, Majersik J, Barsan W, Morgenstern L. Local TV News Reporting of Stroke: A National Perspective. Stroke. 2006;37:621. Ovid Full Text Library Holdings Abstract. [Context Link] 6. Saver JL, Kidwell C, Eckstein M, Starkman S. Prehospital neuroprotective therapy for acute stroke: results of the Field Administration of Stroke Therapy-Magnesium (FAST-MAG) pilot trial. Stroke. 2004;35:e106–e108. Ovid Full Text Bibliographic Links Library Holdings [Context Link] 7. Crocco T, Gullett T, Davis SM, Flores N, Sauerbeck L, Jauch E, Threkeld B, Pio B, Ottaway M, Pancioli A, Chenier T. Feasibility of neuroprotective agent administration by prehospital personnel in an urban setting. Stroke. 2003;34:1918–1922. Ovid Full Text Bibliographic Links Library Holdings [Context Link]
-
"Rid," I posted this because we all hear about it but never actually see it...I thought it may be beneficial for the newer folks to 'see' what in very remote instances could happen...I updated the other thread for ya as well... ACE
-
Should be fixed now.... Manual Chest Compression vs Use of an Automated Chest Compression Device During Resuscitation Following Out-of-Hospital Cardiac Arrest Here's a great study for you all to read.... [quote="Vol. 295 No. 22, June 14, 2006 Featured Link • E-mail Alerts Original Contribution Manual Chest Compression vs Use of an Automated Chest Compression Device During Resuscitation Following Out-of-Hospital Cardiac Arrest A Randomized Trial Al Hallstrom, PhD; Thomas D. Rea, MD, MPH; Michael R. Sayre, MD; James Christenson, MD; Andy R. Anton, MD; Vince N. Mosesso, Jr, MD; Lois Van Ottingham, BSN; Michele Olsufka, RN; Sarah Pennington, RN; Lynn J. White, MS; Stephen Yahn, EMT-P; James Husar, EMT-P; Mary F. Morris; Leonard A. Cobb, MD JAMA. 2006;295:2620-2628. "]ABSTRACT Context High-quality cardiopulmonary resuscitation (CPR) may improve both cardiac and brain resuscitation following cardiac arrest. Compared with manual chest compression, an automated load-distributing band (LDB) chest compression device produces greater blood flow to vital organs and may improve resuscitation outcomes. Objective To compare resuscitation outcomes following out-of-hospital cardiac arrest when an automated LDB-CPR device was added to standard emergency medical services (EMS) care with manual CPR. Design, Setting, and Patients Multicenter, randomized trial of patients experiencing out-of-hospital cardiac arrest in the United States and Canada. The a priori primary population was patients with cardiac arrest that was presumed to be of cardiac origin and that had occurred prior to the arrival of EMS personnel. Initial study enrollment varied by site, ranging from late July to mid November 2004; all sites halted study enrollment on March 31, 2005. Intervention Standard EMS care for cardiac arrest with an LDB-CPR device (n = 554) or manual CPR (n = 517). Main Outcome Measures The primary end point was survival to 4 hours after the 911 call. Secondary end points were survival to hospital discharge and neurological status among survivors. Results Following the first planned interim monitoring conducted by an independent data and safety monitoring board, study enrollment was terminated. No difference existed in the primary end point of survival to 4 hours between the manual CPR group and the LDB-CPR group overall (N = 1071; 29.5% vs 28.5%; P = .74) or among the primary study population (n = 767; 24.7% vs 26.4%, respectively; P = .62). However, among the primary population, survival to hospital discharge was 9.9% in the manual CPR group and 5.8% in the LDB-CPR group (P = .06, adjusted for covariates and clustering). A cerebral performance category of 1 or 2 at hospital discharge was recorded in 7.5% of patients in the manual CPR group and in 3.1% of the LDB-CPR group (P = .006). Conclusions Use of an automated LDB-CPR device as implemented in this study was associated with worse neurological outcomes and a trend toward worse survival than manual CPR. Device design or implementation strategies require further evaluation. Trial Registration clinicaltrials.gov Identifier: NCT00120965 INTRODUCTION Jump to Section • Top • Introduction • Methods • Results • Comment • Conclusion • Author information • References Out-of-hospital cardiac arrest claims hundreds of thousands of lives annually in North America. Successful resuscitation depends on a coordinated set of actions including early cardiopulmonary resuscitation (CPR). High-quality CPR may be important for both cardiac and brain resuscitation.1-3 In animal investigations, fewer interruptions of CPR before and after defibrillation have improved cardiac and neurological outcomes.4-7 The order of resuscitation interventions may also be important, eg, survival may be improved by performing CPR by emergency medical services (EMS) personnel prior to defibrillation.8-9 Observations of rescue personnel indicate that maintaining consistent compressions is a difficult task.10 In the laboratory, trained paramedics provide shallower and slower compressions over time without noticing.11-12 Chest compressions often do not achieve guideline recommendations with regard to depth, rate, and hands-off time.13-14 The desire to provide optimal chest compressions led to the development of automated mechanical chest compression devices. The AutoPulse Resuscitation System (ZOLL Circulation, Sunnyvale, Calif) is a load-distributing band (LDB) circumferential chest compression device with an electrically actuated constricting band on a short backboard and has been approved by the US Food and Drug Administration for use in attempted resuscitation of cardiac arrest. In pig models and in-hospital cardiac arrest in humans, this LDB-CPR device produces greater blood flow to the heart and brain than manual CPR by trained individuals or the automated mechanical piston CPR device.15-16 Animal investigation has demonstrated a greater likelihood of neurologically intact survival in prolonged ventricular fibrillation cardiac arrest with LDB-CPR.17 In this study, the AutoPulse Assisted Prehospital International Resuscitation (ASPIRE) trial, we compared LDB-CPR with manual CPR during out-of-hospital cardiac arrest. We hypothesized that 4-hour survival would be greater among patients randomized to LDB-CPR compared with those randomized to manual CPR. Secondary outcomes were survival to hospital discharge and neurological function at hospital discharge. METHODS Jump to Section • Top • Introduction • Methods • Results • Comment • Conclusion • Author information • References Study Design The study was conducted in Calgary, Alberta; Columbus, Ohio; suburbs of Pittsburgh, Pa; Seattle, Wash; and Vancouver, British Columbia. Because of differences in the time course of ethics review and approval and EMS training schedules, initial study enrollment varied by site, ranging from late July to mid November 2004 (Table 1). All sites halted study enrollment on March 31, 2005. View this table: [in this window] [in a new window] [as a PowerPoint slide] Table 1. Clusters and Enrollment by Site Cost and the inconvenience of carrying the LDB-CPR device to episodes at which it would not be assigned dictated the use of cluster randomization with crossover. Clusters were based on an EMS station or group of stations and crossover occurred at specified time intervals (4 weeks to 2 months). The clustering unit was based on a combination of EMS system operational and design considerations to avoid arrival of both response teams assigned to manual CPR and to automated mechanical LDB-CPR. Within a given site, half of the clusters were randomized to the control (manual CPR) and half to the intervention (LDB-CPR) with subsequent alternation between the intervention and the control. The rotation period was chosen partly for convenience of device transfer, to ensure temporal balance during the trial, and to avoid cyclical phenomena.18 The study was conducted under the regulations for emergency exception from informed consent, which require each US site to inform its community of the proposed trial and seek its opinion.19-20 Notification of individuals enrolled in the study is also required, and the community must be informed of the final results. At each site, including the coordinating center, the primary research review board responsible for guidance, review, and oversight of human subjects' protection approved the study. In addition, research review boards at most receiving hospitals also reviewed and approved the study to allow study personnel to provide timely notification to the patient or family. The study also convened an independent data and safety monitoring board consisting of a paramedic, an EMS physician, a biostatistician, and a clinical investigator. As prespecified in the study protocol, the data and safety monitoring board was to review safety and interim progress when approximately one third and two thirds of patients had been enrolled. Population Adults with out-of-hospital cardiac arrest who received attempted resuscitation by a participating EMS agency were enrolled unless an exclusion criterion was present (Figure). Patients treated by EMS subsequently determined to meet exclusion criteria were excluded from the analysis. View larger version (77K): [in this window] [in a new window] [as a PowerPoint slide] Figure. Flow of Participants in Trial CPR indicates cardiopulmonary resuscitation; EMS, emergency medical services; LDB, load-distributing band. *Cost and the inconvenience of carrying the LDB-CPR device to episodes at which it would not be assigned dictated the use of cluster randomization with crossover. Within a given site, half of the clusters were randomized to the control (manual CPR) and half to the intervention (LDB-CPR) with subsequent alternation between the intervention and the control. Some patients had more than 1 exclusion. A primary comparison population, patients who were in cardiac arrest at the time of EMS arrival and whose cardiac arrest was considered to be of cardiac origin, was chosen a priori as the population most likely to benefit from chest compressions. Cardiac etiology was determined by the site study coordinator or investigator based on the EMS report forms and hospital records. Early in the enrollment, study adherence (application of the LDB-CPR device based on out-of-hospital report) was very low at site D when the advanced life support unit arrived before the study unit. Arrival of an advanced life support unit 90 seconds or longer before the study unit was added as a site-specific exclusion from the primary comparison population. Study Protocol and Intervention The device used in this study is approved by the US Food and Drug Administration and is the size of a half backboard, weighs 15.8 kg, and operates for longer than 60 minutes on battery power. To use the device, the patient's upper body clothing is removed, and the patient is placed supine on the backboard. An 8-inch wide LDB anchored behind the backboard is wrapped circumferentially around the patient's chest and is closed anteriorly with Velcro. The device automatically adjusts the length of the belt so that it fits snugly across the chest. Device-regulated, repetitive shortening of the belt squeezes the thoracic cavity, generating arterial circulation. Belt lengthening enables passive decompression of the chest. The device provides compressions at a rate of 80/min configured as 15 compressions with a 3-second pause for ventilations or continuous chest compressions without any ventilatory pause. During a run-in period ranging from 0.7 to 2.8 months, EMS personnel integrated the automated device into out-of-hospital care. Initial training of EMS personnel included hands-on skill practice using the device with a mannequin and a video presentation with rationale for the LDB-CPR device. Refresher training was not specified by design to best replicate real-world conditions and was highly variable during the study. The protocol allowed 3 options for the resuscitation intervention. Initially all sites chose option 1, a "quick look (<6 seconds)" rhythm recording followed by circulatory effort with either the LDB-CPR device (as soon as ready) or manual compressions by randomized assignment. After approximately 2 minutes or 200 manual compressions, a rhythm assessment was performed. Option 2 was immediate CPR with manual compressions regardless of randomization until the first shock assessment. Site C, the only EMS with a comprehensive quality-improvement effort to reduce pauses in chest compressions, changed its resuscitation intervention to option two 110 days after starting the study. The change was implemented after quality-improvement review identified prolonged time without compressions while deploying the LDB-CPR device. Option 3 allowed analysis, and shock if appropriate, before beginning CPR. In all cases, after rhythm assessment and shock if indicated, additional necessary compressions were to be performed manually or with the LDB-CPR device according to randomization. In all other aspects, sites followed their standard resuscitation protocol until the patient was declared dead or regained stable spontaneous circulation and was transported to and arrived at the emergency department. End Points The primary end point was defined as survival with spontaneous circulation 4 hours after the 911 call. This measure avoids inherent inconsistencies in site-to-site variations in the definition of "admittance to the hospital." Secondary end points included discharge from the hospital and cerebral performance category score at discharge from the hospital that was obtained from the hospital records.21 Data Collection Data were collected from EMS reports, defibrillator recordings, a study questionnaire, and hospital records.22 Study personnel reviewed the defibrillator's digital electrocardiographic recording when available; otherwise electrocardiographic paper strips were reviewed. In addition, an experienced arrhythmia research nurse at the data coordinating center reviewed initial electrocardiographic records. If that review resulted in a discrepancy, the principal investigators, masked to treatment assignment, categorized the initial rhythm. Data collected from the hospital record were primarily used to monitor for adverse effects of chest compression and for ascertaining end points. Sample Size For sample size calculation, patients were assumed to be independent, although the crossover within cluster potentially could be more efficient.23 The 4-hour survival rate was assumed to be the average of the baseline admittance and discharge survival rates. Based on available data from participating sites, we estimated survival to 4 hours (primary outcome) in the manual CPR group to be 17.8%. Based on reported improvements in restoration of spontaneous circulation rates from a small observational study of the device, a 35% relative improvement was hypothesized (ie, an intervention group primary outcome rate of 24.0%).24 Thus, the study required 1837 patients in the primary comparison group to achieve a power of 90% using a 2-sided test with a level of .05 (based on sequential monitoring, 2 interim looks, and an O’Brien-Fleming type boundary).25 The design included the possibility of increasing the sample size based on the observed outcomes to that point.26 Statistical Analysis Comparisons were made by intention-to-treat assignment. Logistic regression was applied using generalized linear mixed models with the robust sandwich estimator of the variance to compare the outcome of individual episodes between the 2 study groups.27-30 Models were adjusted for covariates previously demonstrated to predict survival31-32 as well as cluster (a source of nonindependence). A single a priori subgroup analysis of the primary population was specified based on initial rhythm (asystole, ventricular fibrillation/ventricular tachycardia, pulseless electrical activity). In 6.1% (47/767) of participants, electrocardiographic rhythm was not available and the automated external defibrillator did not advise to shock. These were assumed to be asystole or pulseless electrical activity. Three of the 47 cases were assigned the rhythm observed at the next electrocardiographic analysis. In the remaining 44 cases, the initial rhythm was imputed based on factors that discriminated significantly between patients with initial rhythm of pulseless electrical activity and asystole. Post hoc subgroup analyses evaluated whether the intervention effect differed by site or by the time since the site began enrolling patients. Interactions were tested using an interaction term between treatment group and the covariate of interest. Analyses were conducted using SPSS version 12.0 (SPSS, Chicago, Ill) and R version 2.3 (R Foundation for Statistical Computing) statistical software. Unless explicitly stated, P values are unadjusted for covariates or clustering. For the primary and secondary end points, P values were generally adjusted; by protocol, the level for the primary end point was set at .05. RESULTS Jump to Section • Top • Introduction • Methods • Results • Comment • Conclusion • Author information • References The data and safety monitoring board met on March 11, 2005, and again on March 28, 2005, to review the results for 757 patients enrolled through January 31, 2005, and recommended suspension of enrollment until data for the 314 patients enrolled during February and March could be evaluated. Results prompted additional data collection, including estimates of chest compression duration in the first 5 minutes of the resuscitation effort, drugs administered prior to the patient arriving at the hospital, mode of in-hospital death, and other details indicating lung, heart, or cerebral damage. On June 27, 2005, the steering committee reviewed these expanded data and recommended that the trial be halted. There were 51 clusters, and the average number of episodes per cluster per rotation interval ranged from 1.8 to 25 (Table 1). The number of patient episodes enrolled at sites varied from 120 to 391. There were a total of 1377 episodes, of which 373 in the manual CPR group and 394 in the LDB-CPR group were eligible for study enrollment (Figure). Demographic features, cardiac arrest circumstances, and treatment characteristics were generally similar between the treatment groups. Among primary cases, patients in the LDB-CPR group were more likely to receive epinephrine (P = .03) and have longer time intervals to first shock (for patients found in ventricular fibrillation/ventricular tachycardia) (P = .001), termination of resuscitative effort (P = .01), and hospital transport (P = .01) (Table 2). In the LDB-CPR group, the device was applied during the resuscitation to 83.8% of the primary cases, 73.5% of noncardiac cause cases, and 52.5% of cases for which cardiac arrest occurred after EMS arrival. Among primary study patients, the mean (SD) time from 911 call to first use of the LDB-CPR device was 11.9 (4.5) minutes with a median of 10.9 minutes. View this table: [in this window] [in a new window] [as a PowerPoint slide] Table 2. Episode Characteristics by Primary Case Status and Treatment Group* There was no significant difference in survival at 4 hours after the 911 call between the manual CPR group and the automated LDB-CPR group overall (N = 1071; 29.5% vs 28.5%; P = .74) or among the primary study population (n = 767; 24.7% vs 26.4%; P = .62). Survival to hospital discharge was lower in the LDB-CPR group among primary episodes (5.8% vs 9.9% [P = .04]; adjusted for covariates and clustering, P = .06), but similar among the nonprimary cases (10.6% vs 11.9%; P = .72). Excluding 5 survivors with incomplete neurological data, survival with a cerebral performance category score of 1 or 2 was recorded in 7.5% (28/371) of patients in the manual CPR group compared with 3.1% (12/391) in the LDB-CPR group (P = .006). The survival effect of the LDB-CPR device differed, but not significantly (P = .37), according to initial rhythm of ventricular fibrillation, pulseless electrical activity, or asystole. In contrast to the ventricular fibrillation and pulseless electrical activity subgroups, outcomes trended better in the LDB-CPR group in the asystole subgroup for 4-hour survival (17.2% vs 10.4%) and hospital discharge (1.7% vs 0.6%) (Table 3). View this table: [in this window] [in a new window] [as a PowerPoint slide] Table 3. Outcome by Treatment Group Overall and by Rhythm Subgroup Among Primary Comparison Population* The results of the logistic regression analysis of hospital survival for the primary comparison cases are shown in Table 4. Traditional risk factors for unsuccessful resuscitation (older age, unwitnessed collapse, longer response time, nonpublic location, and initial rhythm of asystole or pulseless electrical activity) were confirmed. The association between hospital survival and treatment group did not differ over time from the start of the study at each site (P = .84 for interaction, adjusted for other covariates and clustering). View this table: [in this window] [in a new window] [as a PowerPoint slide] Table 4. Logistic Regression of Survival to Hospital Discharge* As expected from historical rates, survival was significantly better in site C compared with other sites (Table 4 and Table 5). However, the association between survival and treatment group did not differ significantly at site C compared with the other sites (P = .12 for interaction, adjusted for other covariates and clustering; Table 4). Both before and after the December 28 protocol change, EMS personnel at site C had higher protocol compliance and used the LDB-CPR device earlier in the resuscitative effort than EMS personnel at the other sites (Table 5). View this table: [in this window] [in a new window] [as a PowerPoint slide] Table 5. Comparison of Site C With Other 4 Sites for Primary Cases* A post hoc multivariable analysis, which focused on patients who were treated relatively quickly after their cardiac arrest (witnessed primary cases found in ventricular fibrillation or pulseless electrical activity), indicated that as first vehicle response time shortened, patients in the manual CPR group were increasingly more likely to survive to hospital discharge compared with patients in the LDB-CPR group (interaction P = .06). At 6.6 minutes of response time, the model indicated the treatment groups would have the same survival. Mode of death in the hospital was similar between the treatment groups. Approximately 35% died within 48 hours from a presumed cardiac cause. COMMENT Jump to Section • Top • Introduction • Methods • Results • Comment • Conclusion • Author information • References In this trial comparing manual CPR with automated LDB-CPR, interim results prompted early termination as recommended by the data and safety monitoring board. Although 4-hour survival was similar between treatment groups among primary cardiac arrest episodes, hospital discharge survival was lower in the LDB-CPR group (5.8% vs 9.9%) as was survival with intact neurological status. Evidence indicates that increased blood flow during CPR should translate to a higher likelihood of successful resuscitation.2 The LDB-CPR device evaluated in this study produces greater circulation than manual CPR in animal models of cardiac arrest.16-17 In observational human studies of the device, most but not all investigators have indicated greater likelihood of return of spontaneous circulation compared with historical controls, with 1 study demonstrating better survival to hospital discharge.24, 33-34 The results of the current randomized study were not expected and there is no obvious explanation. One potential explanation is that patients in the manual CPR group benefitted from a Hawthorne effect such that manual CPR quality initially exceeded standard practice.35 Conversely, there could have been a "learning curve" for use of the device with performance expected to improve over time. However, during the last 2 months, survival to hospital discharge for primary cases was 8.1% for manual CPR and 5.0% for LDB-CPR, findings similar to those from the initial months of the study (11.7% and 8.0%, respectively). Another possible explanation for the outcomes is that deployment time for the LDB-CPR device was prolonged. Mean time to first shock in primary cases with initial rhythm of ventricular fibrillation occurred 2.1 minutes later in the LDB-CPR group. While device deployment time was not measured directly, site C applied the device earlier and more frequently than the other sites and yet showed greater relative hazard for the intervention (Table 5). Another implementation-based explanation is enrollment bias. Enthusiasm for the automated LDB-CPR device could have motivated EMS personnel to enroll patients who usually would have been declared dead on arrival. This may have occurred in a few cases because 21 more primary patients were enrolled in the LDB-CPR group compared with the manual CPR group. However, almost all long-term survivors were among patients whose initial rhythm was ventricular fibrillation, pulseless ventricular tachycardia, or pulseless electrical activity, and for whom enrollment and baseline characteristics were comparable between the 2 study groups. Moreover, the adverse intervention relationship was seen among patients presenting with ventricular fibrillation, a group that would routinely receive resuscitation and for whom enrollment bias was unlikely. Other potential explanations for our findings may be related to the direct physiological effects of the automated device. Medications administered with superior blood flow generated by the device might exceed therapeutic thresholds and instead be toxic. However, we are unaware of evidence for such an effect. An additional consideration involves the manner in which blood flow is generated (ie, 80 compressions/min with the LDB-CPR device vs manual CPR rates of 100 compressions/min). There may be an as-yet unmapped relationship between time, flow, and reperfusion injury when early low blood flow may generate less reperfusion injury.36-37 There is also the possibility that chest compressions by the LDB-CPR device may cause direct physical damage to the cardiopulmonary system, although review of hospital records to monitor for adverse events did not overtly identify this possibility.38 In addition, there is a 1 in 40 chance that the adverse survival outcome could have occurred under the null hypothesis of no treatment effect. In this regard, the possibility of unequal risk in the groups randomized at site C should be considered. That site accounted for 40% of the survivors, and survival in its manual CPR group was substantially greater than in previous years. The effect of LDB-CPR compression may have differed depending on the presenting rhythm or time from collapse to resuscitation effort. Patients with asystole, potentially most consistent with untreated and longer arrest duration, appeared to benefit from the LDB-CPR compression whereas those with ventricular fibrillation or pulseless electrical activity appeared to experience harm. In a post hoc multivariable analysis of witnessed primary cases found in ventricular fibrillation or pulseless electrical activity, shorter response times favored the manual CPR group, while the model indicated the treatment groups would have the same survival when the response time reached 6.6 minutes (P = .06 for interaction). To some extent, this finding may be interpreted as consistent with other reports of observational human studies that have evaluated this LDB-CPR device.24 These relationships and their underlying mechanisms require additional investigation. Just as poor adherence dilutes the observed effect of a beneficial treatment, it also dilutes the effect of a harmful treatment. Thus, the observed differences between site C and the other sites are compatible with the overall impression that this implementation of mechanical CPR with the LDB-CPR device may be harmful. The differences are also compatible with the concept that the magnitude of harm may depend on the capabilities of the EMS system. This study has several limitations. The LDB-CPR device was implemented at various stages of resuscitation, a flexibility designed to minimize CPR interruptions. A protocol requiring device implementation at a particular point of care might produce different results. For example, device application in apparently late stages of arrest (the asystole subgroup) appeared to be modestly beneficial. Although each site conducted a run-in phase with the device, more intensive training or a longer run-in phase may have produced different results. The study evaluated the proportion of time with CPR during the first 5 minutes of EMS resuscitation, but did not evaluate the "quality" of manual CPR (ie, rate, depth, recoil) or how manual and LDB-CPR compression differed later in the course of resuscitation. Because of adverse trends in safety outcomes, the study was terminated prior to complete enrollment. Although stopping the study for statistical futility was not part of the prespecified monitoring plan, the conditional power to detect the hypothesized difference in the primary outcome was only 0.55 at the time of study termination. CONCLUSION Jump to Section • Top • Introduction • Methods • Results • Comment • Conclusion • Author information • References As implemented in this study, the use of an automated LDB-CPR device for resuscitation from out-of-hospital cardiac arrest appeared to result in lower survival and worse neurological outcomes than traditional manual CPR. Device design and implementation strategies may need further preclinical evaluation. The results of this study underscore the complexity of resuscitation from out-of-hospital cardiac arrest. Further research is required to understand the interaction of manual or assisted chest compressions with other aspects of resuscitation such as the phase of the arrest,39 drug choice and dose, timing of defibrillation, and treatments such as hypothermia and coronary reperfusion. AUTHOR INFORMATION Jump to Section • Top • Introduction • Methods • Results • Comment • Conclusion • Author information • References Corresponding Author: Al Hallstrom, PhD, Department of Biostatistics, University of Washington, 1107 NE 45th St, Suite 505, Seattle, WA 98105 (aph@u.washington.edu). Author Contributions: Dr Hallstrom had full access to all of the data in the study and takes responsibility for the integrity of the data and the accuracy of the data analysis. Study concept and design: Hallstrom, Rea, Sayre, Christenson, Anton, Mosesso, Van Ottingham, Cobb. Acquisition of data: Rea, Sayre, Christenson, Anton, Mosesso, Olsufka, Pennington, White, Yahn, Husar, Cobb. Analysis and interpretation of data: Hallstrom, Rea, Sayre, Christenson, Anton, Mosesso, Van Ottingham, Morris, Cobb. Drafting of the manuscript: Hallstrom, Rea, Sayre. Critical revision of the manuscript for important intellectual content: Hallstrom, Rea, Sayre, Christenson, Anton, Mosesso, Van Ottingham, Olsufka, Pennington, White, Yahn, Husar, Morris, Cobb. Statistical analysis: Hallstrom, Morris. Obtained funding: Hallstrom. Administrative, technical, or material support: Hallstrom, Van Ottingham. Study supervision: Hallstrom, Rea, Sayre, Christenson, Anton, Mosesso, Van Ottingham, Olsufka, Pennington, White, Yahn, Husar, Cobb. Financial Disclosures: Dr Mosesso reported receiving equipment and supplies for the AED clinical trial from the ZOLL Corporation. Funding/Support: This study was sponsored by Revivant Corporation, now part of the ZOLL Corporation. Role of the Sponsor: The funding organization provided the automated devices, assisted with training, and attended some of the investigator meetings, but had no role in the design and conduct of the study; collection, management, analysis, and interpretation of the data; and preparation or approval of the manuscript. The sponsor was provided a 1-month period to review and comment on the manuscript prior to submission. The investigators were under no obligation to incorporate any such input. Acknowledgment: The data and safety monitoring board consisted of Lawrence Brown, EMT-P, Department of Emergency Medicine, Upstate Medical University, Syracuse, NY; David Goff, Jr, MD, PhD, Wake Forest University School of Medicine, Winston-Salem, NC; Joel Singer, PhD, St Paul's Hospital, Vancouver, British Columbia; and Terence Valenzuela, MD, University of Arizona College of Medicine, Tucson. Each data and safety monitoring board member received a fixed amount of money ($3000) independent of how many times the board would need to meet. We acknowledge the dedicated efforts of the following emergency medical service agencies and their personnel: British Columbia Ambulance Service, Baldwin EMS, Calgary EMS, Eastern Area Prehospital Services, McKeesport Ambulance Rescue Service, Medical/Rescue Team South Authority, Northwest EMS, Ross-West View EMS Authority, Seattle Fire Department, Tri-Community South EMS, Columbus Division of Fire EMS, Upper Arlington Fire Division, and Worthington Fire and EMS. Author Affiliations: Departments of Biostatistics (Dr Hallstrom and Mss Van Ottingham and Morris) and Medicine (Drs Rea and Cobb and Ms Olsufka), University of Washington, Seattle; Department of Emergency Medicine, Ohio State University, Columbus (Dr Sayre and Ms White); British Columbia Ambulance Service, Vancouver (Dr Christenson); Calgary Emergency Medical Services, Calgary, Alberta (Dr Anton and Mr Yahn); Department of Emergency Medicine (Dr Mosesso and Mr Husar), School of Medicine, University of Pittsburgh, Pittsburgh, Pa; and St Paul's Hospital, Vancouver, British Columbia (Ms Pennington). REFERENCES Jump to Section • Top • Introduction • Methods • Results • Comment • Conclusion • Author information • References 1. Stiell I, Nichol G, Wells G, et al. Health-related quality of life is better for cardiac arrest survivors who received citizen cardiopulmonary resuscitation. Circulation. 2003;108:1939-1944. FREE FULL TEXT 2. Ewy GA. Cardiocerebral resuscitation: the new cardiopulmonary resuscitation. Circulation. 2005;111:2134-2142. FREE FULL TEXT 3. Gallagher EJ, Lombardi G, Gennis P. Effectiveness of bystander cardiopulmonary resuscitiation and survival following out-of-hospital cardiac arrest. JAMA. 1995;274:1922-1925. ABSTRACT 4. Niemann JT. Cardiopulmonary resuscitation. N Engl J Med. 1992;327:1075-1080. ISI | PUBMED 5. Yu T, Weil MH, Tang W, et al. Adverse outcomes of interrupted precordial compression during automated defibrillation. Circulation. 2002;106:368-372. FREE FULL TEXT 6. Kern KB, Hilwig RW, Berg RA, Sanders AB, Ewy GA. Importance of continuous chest compressions during cardiopulmonary resuscitation: improved outcome during a simulated single lay-rescuer scenario. Circulation. 2002;105:645-649. FREE FULL TEXT 7. Steen S, Liao Q, Pierre L, Paskevicius A, Sjoberg T. The critical importance of minimal delay between chest compressions and subsequent defibrillation: a haemodynamic explanation. Resuscitation. 2003;58:249-258. FULL TEXT | ISI | PUBMED 8. Cobb LA, Fahrenbruch CE, Walsh TR, et al. Influence of cardiopulmonary resuscitation prior to defibrillation in patients with out-of-hospital ventricular fibrillation. JAMA. 1999;281:1182-1188. FREE FULL TEXT 9. Wik L, Hansen TB, Fylling F, et al. Delaying defibrillation to give basic cardiopulmonary resuscitation to patients with out-of-hospital ventricular fibrillation: a randomized trial. JAMA. 2003;289:1389-1395. FREE FULL TEXT 10. Huseyin TS, Matthews AJ, Wills P, O'Neill VM. Improving the effectiveness of continuous closed chest compressions: an exploratory study. Resuscitation. 2002;54:57-62. FULL TEXT | ISI | PUBMED 11. Ochoa FJ, Ramalle-Gomara E, Lisa V, Saralegui I. The effect of rescuer fatigue on the quality of chest compressions. Resuscitation. 1998;37:149-152. FULL TEXT | ISI | PUBMED 12. Hightower D, Thomas SH, Stone CK, Dunn K, March JA. Decay in quality of closed-chest compressions over time. Ann Emerg Med. 1995;26:300-303. ISI | PUBMED 13. Abella BS, Alvarado JP, Myklebust H, et al. Quality of cardiopulmonary resuscitation during in-hospital cardiac arrest. JAMA. 2005;293:305-310. FREE FULL TEXT 14. Wik L, Kramer-Johansen J, Myklebust H, et al. Quality of cardiopulmonary resuscitation during out-of-hospital cardiac arrest. JAMA. 2005;293:299-304. FREE FULL TEXT 15. Timmerman S, Cardoso LF, Ramires JA. Improved hemodynamics with a novel chest compression device during treatment of in-hospital cardiac arrest. Prehosp Emerg Care. 2003;7:162. 16. Halperin HR, Paradis NA, Omato JP. Improved hemodynamics with a novel chest compression device during a porcine model of cardiac arrest. Circulation. 2002;106:538. FREE FULL TEXT 17. Ikeno F, Kaneda H, Hongo Y, et al. Augmentation of tissue perfusion by a novel compression device increases neurologically intact survival in a porcine model of prolonged cardiac arrest. Resuscitation. 2006;68:109-118. FULL TEXT | ISI | PUBMED 18. Hallstrom AP, Paradis NA. Pre-randomization and de-randomization in emergency medical research: new names and rigorous criteria for old methods. Resuscitation. 2005;65:65-69. FULL TEXT | ISI | PUBMED 19. Protection of Human Subjects, 45 CFR 46.116 (1997). 20. Protection of Human Subjects, 21 CFR 50.23 (1979). 21. Herlitz J, Ekstrom L, Wennerblom B, Axelsson A, Bang A, Holmberg S. Prognosis among survivors of prehospital cardiac arrest. Ann Emerg Med. 1995;25:58-63. ISI | PUBMED 22. Jacobs I, Nadkarni V, Bahr J, et al. Cardiac arrest and cardiopulmonary resuscitation outcome reports: update and simplification of the Utstein templates for resuscitation registries: a statement for healthcare professionals from a task force of the International Liaison Committee on Resuscitation (American Heart Association, European Resuscitation Council, Australian Resuscitation Council, New Zealand Resuscitation Council, Heart and Stroke Foundation of Canada, InterAmerican Heart Foundation, Resuscitation Councils of Southern Africa). Circulation. 2004;110:3385-3397. FREE FULL TEXT 23. Connelly LB. Balancing the number and size of sites: an economic approach to the optimal design of cluster samples. Control Clin Trials. 2003;24:544-559. FULL TEXT | ISI | PUBMED 24. Casner M, Andersen D, Isaacs SM. The impact of a new CPR assist device on rate of return of spontaneous circulation in out-of-hospital cardiac arrest. Prehosp Emerg Care. 2005;9:61-67. PUBMED 25. East Version 3.0: Software for the Design, Simulation and Interim Monitoring of Flexible Clinical Trials User Manual. Cambridge, Mass: Cytel Software Corp; 2003. 26. Chen YH, DeMets DL, Lan KK. Increasing the sample size when the unblinded interim result is promising. Stat Med. 2004;23:1023-1038. FULL TEXT | ISI | PUBMED 27. Venables WN, Ripley BD. Modern Applied Statistics With S. 4th ed. New York, NY: Springer Publishing Co; 2002. 28. Rogers WH. Regression standard errors in clustered samples. Stata Tech Bull Rep. 1993;3:88-94. 29. Williams RL. A note on robust variance estimation for cluster-correlated data. Biometrics. 2000;56:645-646. FULL TEXT | ISI | PUBMED 30. Wooldridge JM. Econometric Analysis of Cross Section and Panel Data. Cambridge, Mass: MIT Press; 2002. 31. Vukmir RB. Survival and outcome from prehospital cardiac arrest. http://www.ispub.com/ostia/index.php?xmlFi...n1/survival.xml. Accessibility verified May 15, 2006. 32. Rea TD, Eisenberg MS, Becker LJ, Murray JA, Hearne T. Temporal trends in sudden cardiac arrest: a 25-year emergency medical services perspective. Circulation. 2003;107:2780-2785. FREE FULL TEXT 33. Lairet J, Lee M. A comparison of standard manual cardiopulmonary resuscitation versus the AutoPulse mechanical cardiopulmonary resuscitation device. Ann Emerg Med. 2005;46:S114. FULL TEXT 34. Ornato J. An emergency medical services system resuscitation strategy: using AutoPulseTM as the primary method for delivering chest compressions improves survival from out-of-hospital cardiac arrest (late-breaking trial presentation). Presented at: American Heart Association Resuscitation Science Symposium; November 11-12, 2005; Dallas, Tex. 35. Campbell JP, Maxey VA, Watson WA. Hawthorne effect: implications for prehospital research. Ann Emerg Med. 1995;26:590-594. FULL TEXT | ISI | PUBMED 36. Anderson T, Vanden Hoek TL. Preconditioning and the oxidants of sudden death. Curr Opin Crit Care. 2003;9:194-198. FULL TEXT | PUBMED 37. Blackstone E, Morrison M, Roth MB. H2S induces a suspended animation-like state in mice. Science. 2005;308:518. FREE FULL TEXT 38. Hart AP, Azar VJ, Hart KR, Stephens BG. Autopsy artifact created by the Revivant AutoPulse resuscitation device. J Forensic Sci. 2005;50:164-168. ISI | PUBMED 39. Weisfeldt ML, Becker LB. Resuscitation after cardiac arrest: a 3-phase time-sensitive model. JAMA. 2002;288:3035-3038. FREE FULL TEXT RELATED ARTICLES This Week in JAMA JAMA. 2006;295:2577. FULL TEXT Use of an Automated, Load-Distributing Band Chest Compression Device for Out-of-Hospital Cardiac Arrest Resuscitation Marcus Eng Hock Ong, Joseph P. Ornato, David P. Edwards, Harinder S. Dhindsa, Al M. Best, Caesar S. Ines, Scott Hickey, Bryan Clark, Dean C. Williams, Robert G. Powell, Jerry L. Overton, and Mary Ann Peberdy JAMA. 2006;295:2629-2637. ABSTRACT | FULL TEXT Manual vs Device-Assisted CPR: Reconciling Apparently Contradictory Results Roger J. Lewis and James T. Niemann JAMA. 2006;295:2661-2664. EXTRACT | FULL TEXT THIS ARTICLE HAS BEEN CITED BY OTHER ARTICLES Chest Compression Device Improves Survival in Out-of-Hospital Cardiac Arrest . . . or Does It? Journal Watch Emergency Medicine 2006;2006:7-7. FULL TEXT Device-Assisted Out-of-Hospital CPR: Two Studies Journal Watch Cardiology 2006;2006:4-4. FULL TEXT Manual vs Device-Assisted CPR: Reconciling Apparently Contradictory Results Lewis and Niemann JAMA 2006;295:2661-2664. FULL TEXT
-
(Emergency Medicine Journal 2006;23:578-579; doi:10.1136/emj.2006.035907 © 2006 by BMJ Publishing Group Ltd @ and British Association for Accident and Emergency Medicine REFLECTIONS ON PREHOSPITAL CARE EtCO2: the key to effective prehospital ventilation R Owen1 and N Castle2 1 Surrey Ambulance Service NHS Trust, The Horseshoe, Bolters Lane, Banstead, SM7 2AS, UK 2 Durban Institute of Technology, Durban, South Africa Correspondence to: Corresponding author: R Owen Surrey Ambulance Service NHS Trust, The Horseshoe, Bolters Lane, Banstead, SM7 2AS, UK; robert.owen@surrey-ambulance.nhs.uk Accepted for publication 10 March 2006) DESCRIPTION An advanced life support (ALS) unit staffed by two paramedics and two students was dispatched to a road traffic accident in South Africa. On arrival the team noted an adult male who had fallen from a minibus travelling at speed. The patient was noted to be in extremis: A. Airway was partially obstructed by blood/vomit, although the patient was lying in a lateral position B. Respiration was shallow at 6 breaths min–1 and oxygen saturation via pulse oximetry was 100% on high-flow oxygen C. Heart rate was 100 bpm and blood pressure 160/100 mm Hg D. Eyes – 1; verbal – 1; motor – 3 E. Isolated head injury with no other obvious injuries Treatment priorities where dictated by the need to secure/protect the airway, concerns over hypoventilation,1 and prolonged hospital transfer time. The decision was made to attempt to reduce secondary hypoxic brain injury through endotracheal intubation.2 Due to the presence of trismus, midazolam (5 mg IV) was selected to facilitate intubating conditions. Sedation assisted intubation is common practice in South Africa. Pre-oxygenation was continued with the patient on his side, a second IV line was secured, and the medication and equipment were prepared. Following a thorough but quick team briefing, the patient was log rolled onto his back and intubated. It was decided to hand ventilate the patient until he was in the ambulance where the mechanical transport ventilator would be used. THE ISSUE The authors were aware of the tendency of health care professionals to hyperventilate intubated patients3,4 and therefore one paramedic supervised the ventilation of the patient by a student who was specifically instructed to ensure that the ventilation rate did not exceed 10 min–1. Our aim was to maintain optimum EtCO2, but despite every effort to control hand ventilation, marked variations in the patient’s EtCO2 were noted (range 7–44 mm Hg). These were significantly outside the target range of 32–38 mm Hg that would indicate normocapnia.5 Once ventilation was transferred to the mechanical ventilator, which was set at 10 min–1 with a tidal volume of approximately 6 ml/kg,5 a steady EtCO2 was maintained. On reflection, the team challenged the decision to delay mechanical ventilation until the patient was in the ambulance. REFLECTION The purpose of reflection is to critically review an action and challenge clinical decisions, thereby confirming or changing practice.6 The authors were aware of the deficiencies of hand ventilation compared to the use of mechanical ventilation7 but decided to delay use of the ventilator until the patient was in the ambulance to facilitate patient movement. This decision was based on the belief that careful hand ventilation with EtCO2 monitoring would allow an acceptable EtCO2 range to be maintained. Without reflective practice there is a risk that clinicians will continue to act on "auto-pilot" as was the case in this instance.8 It is common practice to hand ventilate a patient until a mechanical ventilator has been configured or during short transfers, and prior to this case the authors had not challenged this mode of ventilation as it had become an accepted standard of care. It is evident from the literature that hyperventilation occurs during the prehospital management of intubated patients3,4 and that this has a negative impact on outcomes.4 Our reflection highlighted the inability of hand ventilation to achieve effective ventilation as there is no control of minute volume due to breath-to-breath variability.9 However, variations of up to 20% from the pre-set values of the commonly used transport ventilators have been reported,9 but this difference will remain constant and therefore can be detected and compensated for. A key aspect of prehospital ventilation as demonstrated during our reflection is patient specific tidal volumes, thereby challenging the previous approach of 10 ml/kg at 10 min–1.10 The choice of 6 ml/kg at 10 min–1 facilitated effective ventilation as demonstrated by Helm et al.11 The management of this patient inadvertently provided an opportunity to make a qualitative and quantitative comparison of two ventilation strategies. This case highlighted the role of EtCO2 monitoring in the context of prehospital ventilation and the importance of education in ventilation strategies and not just the psychomotor skill of intubation. Perhaps most importantly for the authors, it provided stark evidence that patient care can be improved by challenging commonly held beliefs. The reflection that followed this case has resulted in a number of changes to the standard operating procedures of the paramedic unit involved, resulting in the earlier use of mechanical ventilation and mandatory use of EtCO2 in all intubated patients. This is particularly important, as this ALS unit has been tasked with evaluating the introduction of prehospital rapid sequence intubation within South Africa. FOOTNOTES Competing interests: none declared REFERENCES Dunham MC, Barraco RD, Clark DE, et al. Guidelines for emergency tracheal intubation immediately after traumatic injury. J Trauma 2003;55:162–79.[Medline] Winchell RJ, Hoyt DB. Endotracheal intubation in the field improves survival in patients with severe head injury. Arch Surg 1997;132:592–7.[Abstract] Aufderheide TP, Sigurdsson G, Pirrallo RG, et al. Hyperventilation-induced hypotension during cardiopulmonary resuscitation. Circulation 2004;109:1960–5.[Abstract/Free Full Text] Davis DP, Dunford JV, Poste JC, et al. The impact of hypoxia and hyperventilation on outcome after paramedic rapid sequence intubation of severely head-injured patients. J Trauma 2004;57:1–10.[Medline] Rayner-Klein J. Commentary on: pre-hospital emergency anaesthesia. J R Army Med Corps 2004;150:72–3. Johns C. Becoming a reflective practitioner. A reflective and holistic approach to clinical nursing, practise development and clinical supervision. London: Blackwell Science, 2000. Helm M, Hauke J, Lampl L. A prospective study of the quality of pre-hospital emergency ventilation in patients with severe head injury. B J Anaesth 2002;88:345–9.[Abstract/Free Full Text] Cox H, Hickson P, Taylor B. Exploring reflection: knowing and constructing practice. In: Gray G, Pratt R, eds. Towards a discipline of nursing. Edinburgh: Churchill Livingstone, 1994:378. Helm M, Fischer S. The role of capnography in pre-hospital ventilation for trauma patients. Int J Intensive Care 2005;12 (3) :124–30. Walls RM, Luten RC, Murphy MF, et al, eds. Manual of emergency airway management. Philadelphia: Lippincott, Williams and Wilkins, 2000. Helm M, Schuster R, Hauke J, et al. Tight control of prehospital ventilation by capnography in major trauma victims. B J Anaesth 2003;90:327–32.[Abstract/Free Full Text]
-
Intracranial placement of nasopharyngeal airways: is it all that rare? (Emergency Medicine Journal 2006;23:661; doi:10.1136/emj.2006.036541 © 2006 by BMJ Publishing Group Ltd @ and British Association for Accident and Emergency Medicine LETTER Intracranial placement of nasopharyngeal airways: is it all that rare? D Y Ellis, C Lambert and P Shirley Intensive Care Department, Royal London Hospital, London, UK Correspondence to: D Y Ellis Intensive Care Department, Royal London Hospital, London, UK; danellis@doctors.org.uk) In their recent review on nasopharyngeal airways (NPA), Roberts et al1 mention that the "evidence for avoiding NPAs in case of basal skull fracture is based solely on two case reports".2,3 This may be true, but how many instances of intracerebral NPA placement occur but are not published? Once a complication has occurred and been reported in a journal, further similar case reports are less likely to be submitted because it is no longer a novel complication. We recently had another episode of accidental cerebral misplacement of an NPA in a trauma patient who later proved to have a base of skull fracture. The patient was a coach driver who was involved in a head on collision with a low building and sustained severe facial injuries. Extrication proved complicated, and the patient was trapped in the vehicle for approximately 45 min. His GCS was 3/15 when the ambulance service arrived and it was judged that his airway was at risk. The paramedics were unable to open his mouth and took the decision to insert an NPA. The precise method of insertion is not documented. On arrival in the ED the patient was intubated and ventilated and underwent a CT scan of the brain. This showed multiple facial fractures, including severe disruption to the base of the skull. The NPA was seen to enter the cerebral cortex (fig 1). We fully agree that airway management takes priority in the emergency situation and such advice is especially relevant in this type of patient when a contraindication to NPA placement (base of skull fracture) cannot be excluded clinically. However, it is also likely that suspected base of skull fracture has led to fewer NPAs being sited in patients with any form of head injury, perhaps leading to substandard airway care. In some circumstances, NPA placement may be necessary even when there are relative contraindications and we support the author’s claim that teaching on NPAs should focus on methods of safe placement. However, we would question the authors’ comment that the complication of intracranial tube placement is "oft quoted but very infrequent". FOOTNOTES Informed consent was obtained for publication of the person’s details in this report Competing interests: none declared References Roberts K, Whalley H, Bleetman A. The nasopharyngeal airway: dispelling myths and establishing the facts. Emerg Med J 2005;22 (6) :394–6.[Abstract/Free Full Text] Schade K, Borzotta A, Michaels A. Intracranial malposition of nasopharyngeal airway. J Trauma 2000;49 (5) :967–8.[Medline] Muzzi DA, Losasso TJ, Cucchiara RF. Complication from a nasopharyngeal airway in a patient with a basilar skull fracture. Anesthesiology 1991;74 (2) :366–8.[Medline] Related Article Primary Survey Geoff Hughes Emerg. Med. J. 2006 23: 587. [Extract] [Full Text] (Emerg Med J 2005; 22:394-396 © 2005 BMJ Publishing Group Ltd @ and British Association for Accident and Emergency Medicine REVIEW The nasopharyngeal airway: dispelling myths and establishing the facts K Roberts1, H Whalley2 and A Bleetman3 1 Specialist Registrar in General Surgery, Walsall Manor Hospital 2 Senior house officer in Intensive Care Medicine, Birmingham Heartlands Hospital, UK 3 Consultant in Accident and Emergency Medicine, Birmingham Heartlands Hospital, UK Correspondence to: Keith Roberts dr_keith@hotmail.com Accepted for publication 13 March 2005) ABSTRACT The nasopharyngeal airway (NPA) is a simple airway adjunct used by various healthcare professionals. It has some advantages over the oropharyngeal airway (OPA) but despite this it appears to be used less frequently. This may be due to fears over intracranial placement in cases of possible basal skull fracture. This fear, promulgated by training, is based solely on two single case reports and relative risk needs to be put into clinical context. Widely taught methods of sizing NPAs are based upon the width of the patient’s nostril or little finger, MRI data demonstrate that these methods are inaccurate. Ideal NPA length measured at nasal endoscopy correlates with subject height, this is independent of subject sex, and is a far more accurate determinant and easy to use in the clinical setting. Average height females require a Portex size 6 NPA and average height males a size 7 Portex NPA. This knowledge provides a rapid method of NPA selection. -------------------------------------------------------------------------------- Abbreviations: NPA, nasopharyngeal; OPA, oropharyngeal The nasopharyngeal airway (NPA) is a simple airway adjunct used in a number of healthcare disciplines, by staff trained to varying levels of competence in airway management. It has advantages over the oropharyngeal airway (OPA) as it can be used in patients with an intact gag reflex, trismus or oral trauma. Despite this, it is used less frequently than the OPA.1,2 This may be due to fears over intracranial placement in cases of possible basal skull fracture, promulgated in training. The basis for these concerns is revisited. Widely taught methods of sizing NPAs are based upon the width of the patient’s nostril or little finger.3,4 The accuracy of these methods are reviewed in light of recent evidence. We sought a more accurate and rapid method of sizing NPAs. METHODS A Medline search was conducted with ‘nasopharyngeal airway’ as the key words. RESULTS The results identified 494 abstracts. Papers in English that reviewed indications and/or contraindications to NPA use and those that discussed methods of sizing NPAs were selected for review. The level of evidence for each paper referred to is presented where appropriate. (Level I, Strong evidence from at least one systematic review of multiple well-designed randomised controlled trials. Level II, Strong evidence from at least one properly designed randomised controlled trial of appropriate size. Level III, Evidence from well-designed trials such as non-randomised trials, cohort studies, time series, or matched case-controlled studies. Level IV, Evidence from well-designed non-experimental studies from more than one centre or research group. Level V, Opinions of respected authorities, based on clinical evidence, descriptive studies or reports of expert committees.) DISCUSSION Sizing an NPA Widely taught3,4 methods of sizing an NPA include comparing an NPA to the width of the patients little finger or nares. In paediatric practice it is also taught that an appropriate NPA will blanch the nares.5 In 2002 a study6 (Level III) assessed the validity of assessing subjects’ little fingers or nares in order to size an appropriate NPA. Ten caucasian subjects attending for elective MRI of their skull had an additional sequence of scans performed at right angles to the nasal floor. From these the largest diameter NPA that would fit the subjects was calculated and compared with the subjects’ little fingers and nares (as measured on the scans). The only measurement that closely correlated with the internal nasal anatomy was the medial—lateral distance of the subject’s distal interphalangeal joint. The r-value of association on the right side was 0.02 and on the left 0.11 (values of 1 or –1 indicate complete correlation and 0 no correlation). The other measurements overestimated the appropriate airway in every instance. Therefore the currently taught methods of sizing NPAs are misleading. In 1993, Stoneham7 (Level III) described that a correctly placed NPA will lie just above the epiglottis having separated the soft palate from the posterior wall of the oropharynx. This knowledge is vital if the NPA is to be sized correctly in patients: if the airway is too short it will fail to separate the soft palate from the pharynx and if too long it can either pass into the larynx and aggravate cough and gag reflexes or pass anterior to the epiglottis into the vallecula, a blind ending pouch, where paradoxical airway obstruction can occur if the NPA lumen is pressed against the soft tissues. Stoneham observed that the ideal NPA should lie approximately 10 mm above the epiglottis. Thus the important factor in sizing a NPA is not the width of the tube, but rather the length. In the study Stoneham clearly identified a relationship between nares—epiglottis (N-E) distance and subject height. 120 adult caucasian subjects, under general anaesthesia, had their N-E distance measured. Subject height correlated with this distance and was independent of patient sex. Stoneham’s average height females (163 cm/5' 4'') had an N-E distance of 140 mm (95% CI 136.1–143.5 mm) and males (178 cm/5' 10'') an N-E distance of 159 mm (95% CI 156.1–161.2 mm). Accepting that the airway should lie 10 mm above the epiglottis average height females in this study require a size 6 PortexTM (130 mm long) NPA and similarly average height males a size 7 PortexTM (150 mm long) NPA. Because of the association between subject height and N-E distance a suitable NPA can be rapidly selected – for example, a tall male requires a size 8 NPA and a short male a size 6. A tall female a size 7 and a short female a size 6 with the safety pin placed 1 cm from the flange. A sizing chart based on Stoneham’s work is presented in table 1. The suggestions are based upon the evidence presented but ultimate selection will depend upon clinical response. View this table: [in this window] [in a new window] Table 1 Subject height and suggested NPA size The association between subject height and N-E distance has also been identified in 73 Chinese subjects8 (level III). In this series the male N-E distance was 160 +/– 10 mm and females 150 +/– 9 mm. It appears that this trend also applies to paediatric practice. A study of 413 infants under 12 months also identified an association between subject height and nares-vocal cord distance with an r-value of 0.83 (p = 0.0001)9 (Level III). This study also identified a very close association between nares-vocal cord distance and nose tip-earlobe distance (see table 2). In order to place the NPA in infants the insertion length must therefore be slightly less than this anthropometric measurement. View this table: [in this window] [in a new window] Table 2 Infant weight and suggested insertion length of an appropriate NPA Contraindications and indications for use From reviews of paramedic practice, it is apparent that the use of the NPA outside of hospital is limited. In 2000, a review of national paramedic practice identified that only 27% of UK NHS Ambulance Trusts provide their paramedics with NPA’s10 (Level IV). A further review in 2002, following the publication of national guidelines supporting the use of the NPA, found that this practice had increased, but only to 55%. Similarly in 2003 only 21% of Ambulance Trusts would permit their paramedics to use NPA’s in paediatric care11 (level IV). The scale of NPA use in hospitals is less clear. However, it is the authors’ observation that NPAs are used less frequently than OPAs. It is not clear why this might be the case. They are cheap and easy to use. It is possible that the fear of complications associated with the NPA has limited its use. It is widely taught that a suspected or known basal skull fracture is a contraindication to NPA placement. This is based upon two single case reports12,13 (Level V). This contraindication needs to be interpreted in the appropriate setting: faced with airway obstruction and the possibility of a basal skull fracture a rescuer must secure the airway prior to any further management. In hospitals when a patient is known to have a basal skull fracture it is highly likely that this will have been diagnosed following a CT scan. In this scenario the issue of airway care will already have been addressed. Prior to a CT scan the diagnosis of a basal skull fracture can only be presumed by the presence or absence of clinical features. These include blood or cerebrospinal fluid from the ears or nose and bruising around the mastoid process or eyes. It must be appreciated that blood from the nose of patients with traumatic injuries is very common as is blood in the external ear (which has dripped into the ear rather than from within). CSF is a clear fluid and its identification is difficult, even in hospital. The simplest tests, the halo sign or the presence of glucose, are easy to perform but have a low sensitivity and specificity14,15 (Level III). The gold standard for laboratory diagnosis of CSF is the presence of beta-2-transferrin,14 this however, has no practical value as results are reported in 4 days. Bruising around the mastoid or eyes is also common following trauma and is more likely to be associated with soft tissue injury than a basal skull fracture. Outside of hospital and particularly in low light or wet prehospital conditions, the recognition of CSF from the nose or ears is near impossible. Furthermore the development of this bruising will take some time and is unlikely to have developed when the patient is in the early stages of resuscitation whether this is in the pre-hospital environment or upon arrival to the Emergency Department. Therefore, the clinical features are vague and reliance upon their presence or absence may unnecessarily deter the use of the NPA. The evidence for avoiding NPAs in cases of basal skull fracture is based solely upon two case reports. Securing the airway in an emergency takes precedence over a suspected basal skull fracture. The OPA is an obvious alternative but in practice a patient must have a conscious level much below that at which an NPA will be tolerated. If an OPA is placed and aggravates the gag reflex the risk of vomiting, aspiration, and increased intra-cranial pressure increases. In teaching the use of the NPA, focus needs to shift from fear of contraindications to methods of safe placement to avoid intracranial placement. This needs to emphasise lifting the nares to reveal the nasal airway and the placement of the NPA parallel to the nasal floor, rather than upwards towards to the cribriform plate of the ethmoid bone. Lubrication, gentle rotation of the NPA and trying both nostrils are further methods that will ease insertion. These will reduce any risk of the often quoted but very infrequent complication of intracranial tube placement. Other complications of NPA placement relate to soft tissue damage of the nasal mucosa. The mucosa is lined with pseudostratified columnar epithelium and vascular tissue that is similar to the erectile tissue of the penis. This tissue allows the normal cyclical variation in airway volume and alternates airflow between nostrils. It can be stripped off the nasal wall periosteum, causing bleeding. This is a relatively common problem associated with NPA insertion. If resistance is encountered when placing an NPA it is therefore recommended that the other nostril should be used to minimise this risk. A comparison of nasal soft tissue trauma as a result of NPA placement found no difference between the rates of haemorrhage between nurses or experienced anaethetists16 (Level III). Another rare complication is migration into the nasopharynx of the entire NPA17,18 (Level V), it is for this reason that Portex NPA’s have a flange and are supplied with a safety pin. CONCLUSIONS The NPA is a simple piece of equipment that is easy to use and cheap. It is effective and has advantages over the OPA but appears to be used less frequently. Traditional methods of sizing NPAs place emphasis upon the width of the patient’s nares or little finger, these are inaccurate and must be abandoned; tube length is more important. Evidence clearly demonstrates a relationship between NPA length to subject height, which is independent of sex. Average height females require a size 6 PortexTM NPA and males a size 7. Optimal and rapid sizing of the NPA can be modified from these average sizes to take account of the subject’s height. The widely taught contraindication to NPA placement of a suspected basal skull fracture may be the reason that NPA use is less than that of the OPA. This extremely rare complication must be considered in relation to the potential benefit of simple and effective airway management. Fear of this complication will provide high false positive prediction of the fracture and may dissuade prehospital carers from using a very effective airway adjunct. Selecting an OPA in preference to an NPA in patients with an intact gag reflex risks vomiting and aspiration pneumonia. This maybe as equally grave as intracranial placement of a NPA and is certainly a more commonly encountered problem. Therefore methods of teaching must move from fear of complications to methods of safe placement of simple airway adjuncts and appropriate patient selection. FOOTNOTES Competing interests: none declared KR and AB are both HEMS doctors, Warwickshire and Northamptonshire Air Ambulance REFERENCES Roberts K, Allison KP, Porter KM. A review of emergency equipment carried and procedures performed by UK front line paramedics. Resuscitation 2003;58 (2) :153–8.[CrossRef][Medline] Allison K, Porter K. Nasopharyngeal airways: an under-utilised pre-hospital resource. Pre-Hospital Immediate Care 2000;4 (4) :192–3. Gwinnut C . Lecture notes on clinical anaesthesia, Blackwell Scientific Publications, Oxford 1997:p39. Greaves I, Porter KM, Ryan J. Trauma care manual. Arnold 2001:p41. Advanced Paediatric Life Support Course Manual, BMJ books third ed. 2001:p36. Roberts K, Porter K. How do you size a nasopharyngeal airway? Resuscitation 2003;56 (1) :19–23.[CrossRef][Medline] Stoneham MD. The nasopharyngeal airway. Assessment of position by fibreoptic laryngoscopy. Anaesthesia 1993;48 (7) :575–80.[Medline] Hwang CL, Luu KC, Wu TJ, et al. Estimation of the length of nasopharyngeal airway in Chinese adults. Ma Zui Xue Za Zhi 1990;28 (1) :49–54.[Medline] Shen CM, Soong WJ, Jeng MJ, et al. Nasopharyngeal tract length measurement in infants. Acta Paediatr Taiwan 2002;43 (2) :82–5.[Medline] Porter K, Allison K, Greaves I. Variations in equipment on UK front line. ambulances. Pre-hospital Immediate Care 2000;4:126–131. Roberts K, Jewkes F, Whalley H, et al. Equipment carried and procedures performed by UK paramedics upon paediatric patients. Resuscitation Submitted June 2004. Muzzi DA, Losasso TJ, Cucchiara RF. Complication from a nasopharyngeal airway in a patient with a basilar skull fracture. Anaesthesiology 1991;74:366–8.[Medline] Schade K, Borzotta A, Michaels A. Intracranial malposition of nasopharyngeal airway. J Trauma 2000;49 (5) :967–8.[Medline] Constantino PD, Janecka IP. Cranial-base surgery. In: Head and Neck Surgery—Otolaryngology, second edn. In: Byron J, ed. Bailey. Lipincott-Raven, Philadelphia 1998:1848–1853. Chan DT, Poon WS, IPCP, et al. How useful is glucose detection in diagnosing cerebrospinal fluid leak? The rational use of CT and Beta-2 transferrin assay in detection of cerebrospinal fluid fistula? Asian J Surg 2004;27 (1) :39–42.[Medline] Chung CH, Sum CW, Li HL, et al. Comparison of nasal trauma associated with nasopharyngeal airway applied by nurses and experienced anesthesiologists. Changgeng Yi Xue Za Zhi 1999;22 (4) :593–7.[Medline] Smith BL. Retained nasopharyngeal airway. Anaesthesia 1989;44 (5) :447.[Medline] Milam MG, Miller KS. Aspiration of an artificial nasopharyngeal airway. Chest 1988;93 (1) :223–4.
-
No apologies necessary, I agree, and for the record, I apply the seach comment universally...Jate to be accused of discrimination..but I think you'll find some interesting points and information about this in other threads. Hope this helps, ACE844
-
Doctor and 2 Nurses arrested for possible "euthansia&qu
Ace844 replied to Joshua Benton's topic in General EMS Discussion
"Josh," Here's an article which relates to your query and thought may help you with some more perspective... (Vol. 296 No. 3 @ July 19, 2006 The Physician’s Counsel Donald A. Misch, MD Evanston, Illinois dmisch@northwestern.edu JAMA. 2006;296:259-260. ) More than ten years ago my mother died as a result of a sudden, massive stroke. Although she had suffered from breast cancer, she had been doing well until she made an out-of-state visit to my family. During her visit there was no specific symptom or sign that something was wrong, but my mother simply did not look good, appearing less energetic than usual. Several days after returning home she was found unconscious on the floor, having apparently lain that way for most of the previous night. My mother spent the subsequent two weeks in the hospital. Computed tomography showed enormous bilateral cerebral infarcts with tremendous surrounding edema. No etiology could be determined to account for the extensive tissue destruction on both sides of her brain. What became increasingly obvious was that my mother was not going to recover. She had severe aphasia and motor deficits, and it appeared that she was unaware of events proceeding around her. She was unable to care for herself. And yet, her cancer was, to the best of our knowledge, in remission; her heart was strong; and, with the exception of her brain, she was in generally good health. And so the question arose: What should be done? My mother did not have a written living will or advance directive, but she had made it clear to the family and her oncologist that if her breast cancer had recurred she would commit suicide. She had already made contact with the Hemlock Society, and she was an ardent supporter (too ardent, from my perspective) of Jack Kevorkian and his campaign for assisted suicide. I am the only physician in our immediate family. Thus, my assessment of my mother's situation, my perspective on the likelihood of any significant recovery, and my judgment as to whether every appropriate—a very important word—step had been taken in diagnosis and treatment were to be central in the family's ultimate decisions as to her future care. Our entire family knew what my mother would have wanted, indeed what she would have demanded in her usual way, were she able to speak for herself. Whatever other qualities she may have possessed, she was a woman who never sat still, who never let cancer slow her down, who was determined to die on a golf course where she could drive a golf ball further than many men. And she had told us all, in no uncertain terms, that if she could not continue to enjoy life in exactly that way, she did not want to live. I believe then, and I still do, that my mother would have "pulled her own plug" had she been able to do so. And I, and all of the physicians involved in her care, believed that the likelihood of any significant recovery was nil; the cerebral damage was massive, overwhelming, and irreversible. And so my family and I decided to let nature take its course. But first I needed to speak with a physician, a rheumatologist who had been my personal physician and my friend, who had been asked to consider the possibility of vasculitis as a cause of my mother's illness. When he and I discussed the situation, we both used the same information as to what had happened to my mother, the laboratory tests and CT scan findings, and what we knew of her wishes. Independently we both came to the same conclusion—in line with my mother's wishes, no heroic measures should be undertaken. I did not need another physician to assess the situation intellectually and medically, and yet it was clear that my rheumatologist friend's words were critically important to me. I needed a physician's counsel to let my mother die. This was true even though I was, and am, a physician, dually trained in internal medicine and psychiatry, and I had on many other occasions helped other families struggle with similar decisions. I needed a physician—not simply other family members or friends or advisors (although all of these were helpful as well)—to tell me that under the particular circumstances of my mother's situation, my conclusions were reasonable. Because all decisions about significant others in one's life are laden with emotion, conscious and unconscious meaning, and history, I needed to be sure that my motives for making this irrevocable and lethal decision were not contaminated by my longstanding conflictual relationship with mother. I needed a physician to assure me that my judgment was consistent with my mother's wishes and her best interests. And when my friend, the rheumatologist, told me that under the same circumstances with one of his own parents, he would do the same thing, I cried. I cried partially for the impending death of my mother, but I also cried with relief that I had a physician's counsel in support of such a decision. Physicians make diagnoses and provide treatment. They relieve, or at least ameliorate, suffering, and they help individuals and their families deal with the effects of illness. They give reassurance and hope, and they provide empathy, compassion, and concern. But physicians give something else to patients and patients' families—they give counsel. The Encarta Dictionary defines the noun "counsel" as "advice sought from or given by somebody, especially somebody who is wise or knowledgeable." Similarly, according to the Oxford English Dictionary, "counsel" is an "opinion as to what ought to be done given as the result of consultation; aid or instruction for directing the judgment; advice, direction." When physicians give counsel, they do not substitute their judgment for that of the patient or family. Instead, physicians work in partnership with patients and families to craft a mutually determined decision that best meets the demands of a particular situation. The physician as counselor encourages patients and families to explore their own beliefs, needs, and motives and to exercise their own best judgment. In the process, however, he or she brings to the table extensive medical knowledge about the symptoms, course, treatment, and prognosis of disease as well as an understanding of the experiences of illness, pain and suffering, fear and loss. Moreover, the physician often possesses an intimate knowledge about a given patient and family. And when these assets are marshaled together with a physician's societal license and symbolic role as a healer, the physician is uniquely suited to provide counsel in health matters. Almost 30 years ago, in my second month as an intern in internal medicine, I admitted a patient who had cancer of the prostate that had widely metastasized to bone and peritoneum. He was in considerable pain, and surgical consultants recommended what, at the time, appeared to be the best opportunity for palliation: bilateral orchiectomy. Pain-ridden as he was, it was clear that the idea of testicular removal was anathema to him. Every day the surgeons would come into his room and ask if he were ready to undergo the operation, and every day he would resist, downcast and ashamed, indicating that he still had not made up his mind. The choices were stark. The surgeons were increasingly frustrated and impatient. After watching this process continue over the course of a week, I asked to speak with him, alone, early one evening. I told him how much I sympathized with his difficult predicament, and I did not presume to know the correct answer to his dilemma. If he were interested, however, I would be willing to give him some of my thoughts. He agreed. I told the patient that, if it were me, the first thing I would want to know are some key facts. Is there any reason to believe this procedure will prolong my life? If I decide not to undergo surgery today, can I change my mind and return tomorrow? If I don't have the operation today, will it be less effective a week from now? We discussed these questions as I provided him with the best information available. I then told him that I could never put myself exactly in his shoes, that I would never know precisely what I would do in his situation. "Nonetheless," I said, "if you’d like, I’ll tell you what I think I might do if I had to make your decision." I went on: "Everyone agrees that this surgical procedure will not prolong your life, so its only purpose is palliation, relief of pain. And if you were to go home today, there is no reason you could not come back tomorrow and have the operation, and it will likely be just as helpful, or not, as if you had it today. But when I listen to you and when I watch you, I think that the psychological pain of having your testicles removed at this time is greater than the physical pain of your cancer. If the goal here is palliation—relief of suffering—it seems to me that right now, for you, the proposed treatment is worse than the disease. I believe that if it were me, I’d go home because I’m just not ready to have this operation today and I know that I can change my mind later." Perhaps it was my imagination, and yet I know—I know—that for the first time a look of relief came over his face and he was close to tears. He went home the next morning, forgoing surgery. I do not know if he returned the next day, the next week, or ever. But when I look back over almost 30 years in medicine, when I ask myself what are the things I have done as a physician for which I feel the greatest satisfaction, this is one of several memories that invariably returns to me. And I may be wrong, but I like to think that somewhere, somehow, this terribly distressed man confronted with a frightening and painful choice continues to thank me for providing him counsel to make the decision that he knew, in his heart, was the right one for him. A Piece of My Mind Section Editor: Roxanne K. Young, Associate Editor. -
(Vol. 296 No. 3 @ July 19, 2006 Avian Flu Clue Joan Stephenson, PhD JAMA. 2006;296:272.) Scientists from Hong Kong report new findings that support the hypothesis that some H5N1 avian influenza viruses are particularly deadly to humans because they can trigger a "cytokine storm" that causes the immune system to overreact (Zhou J et al. J Infect Dis. 2006;194:61-70). The researchers infected cultured human immune cells (monocyte-derived macrophages [MDMs]) with human influenza virus or with the H5N1 strain that caused a deadly 1997 outbreak in Hong Kong. They found that the H5N1-infected MDMs produced much higher levels of chemokines, a type of cytokine that directs white blood cells to inflammation sites. In addition, levels of chemokines and chemokine receptors were higher in MDMs from adults than in MDMs from newborns, suggesting that chemokines might be a key to the 1997 outbreak's higher mortality rate among adults. Scientists have suggested that a cytokine storm played a role in the virulence of the 1918 Spanish flu pandemic. Previous research frequently noted severe lung damage in those who died in the 1918 pandemic; similar damage has been found in lung tissue of individuals killed in recent avian flu outbreaks. The strong induction of chemokines and their receptors by avian flu viruses, particularly in adult immune cells, "may account for the severity of H5N1 disease," the researchers noted.
-
Here's a review of a book from the NEJM. I haven't read it but it is on an oft discussed topic here and thought you all may be interested. It is on my list to read an purchase, when i am done I will comment on it.